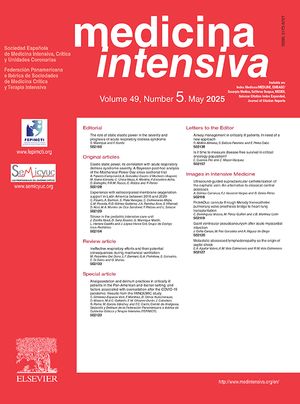
Edited by: Federico Gordo - Medicina Intensiva del Hospital Universitario del Henares (Coslada-Madrid)
Last update: February 2024
More infoAcinetobacter baumannii is a Gram-negative, multidrug-resistant (MDR) pathogen that causes nosocomial infections, especially in intensive care units (ICUs) and immunocompromised patients. A. baumannii has developed a broad spectrum of antimicrobial resistance, associated with a higher mortality rate among infected patients compared with other non-baumannii species. In terms of clinical impact, resistant strains are associated with increases in both in-hospital length of stay and mortality. A. baumannii can cause a variety of infections, especially ventilator-associated pneumonia, bacteremia, and skin wound infections, among others. The most common risk factors for the acquisition of MDR A. baumannii are previous antibiotic use, mechanical ventilation, length of ICU and hospital stay, severity of illness, and use of medical devices. Current efforts are focused on addressing all the antimicrobial resistance mechanisms described in A. baumannii, with the objective of identifying the most promising therapeutic scheme.
El Acinetobacter baumannii es un patógeno Gram negativo, multirresistente (MR) que causa infecciones nosocomiales, especialmente en las Unidades de Cuidados Intensivos (UCIs) y en los pacientes inmunocomprometidos. El A. baumannii ha desarrollado una resistencia antibiótica de amplio espectro, asociada con alta tasa de mortalidad entre los pacientes infectados comparada con las otras especies de Acinetobacter no baumannii. En relación al impacto clínico, las cepas resistentes están asociadas con incremento de la estadía hospitalaria y de la mortalidad. El A. baumannii puede causar diferentes infecciones, especialmente neumonía asociada la ventilación mecánica, bacteriemia, e infección de piel y partes blandas, entre otras. Los factores de riesgo más frecuentes para la adquisición de A. baumannii MR son el uso previo de antibióticos, la asistencia respiratoria mecánica, la estadía en la UCI y en el hospital, la severidad de la enfermedad, y el uso de dispositivos médicos. Los esfuerzos actuales están focalizados en determinar todos los mecanismos de resistencia descriptos en el A. baumannii, con el objetivo de identificar esquemas terapéuticos más efectivos.
The antimicrobial resistance of most microorganisms poses a serious worldwide health threat, increasing costs, prolonging hospital stay and elevating patient mortality. The World Health Organization (WHO) has issued a strong alert on this threat, with the inclusion of carbapenem-resistant Acinetobacter baumannii (AB), carbapenem-resistant Pseudomonas aeruginosa, and extended-spectrum beta-lactamase (ESBL) producing Enterobacteriaceae on the list of “priority 1 (critical) pathogens” that are resistant to multiple antibiotics and constitute a human health hazard. Such pathogens are considered to constitute a priority in the research and development of new antibiotics.1
A recent review carried out in 204 countries established 6 main pathogens associated with multidrug resistance (Escherichia coli, Staphylococcus aureus, Klebsiella pneumoniae, Streptococcus pneumoniae, Acinetobacter baumannii and Pseudomonas aeruginosa), and which were responsible for 929,000 (660,000–1,270,000) attributable deaths and 3.57 million (2.62–4.78 million) deaths associated to multidrug resistance in 2019. The percentage of carbapenem-resistant AB isolates in these countries was 60–80%, and proved particularly high in Asian countries and in regions characterized by low to medium income levels.2
Acinetobacter baumannii is a non-fermenting gram-negative bacterium that causes serious and invasive hospital-acquired infections associated to high mortality. The epidemiological evolution of AB towards multidrug resistance or pan-drug resistance is currently a major challenge that leaves physicians with very few treatment options.3
Mechanisms of antibiotic resistanceThere are three main mechanisms of resistance to antibiotics:
1.- Control of antibiotic (ATB) transport across membranes (reduced porin permeability, increased efflux or decreased ATB influx).
2.- Protection of the bacterium against the effect of the ATB through genetic mutations or post-translation changes that modify the target of the ATB.
3.- Enzymatic inactivation of the ATB through hydrolysis or modification of the ATB.
One of the most important tools of AB for generating resistance is its impressive genetic plasticity, which allows rapid genetic mutation and re-accommodation. Furthermore, AB is able to form biofilms and thus prolong its survival on medical devices such as endotracheal tubes, catheters, etc.3
Resistance to beta-lactams: Acinetobacter baumannii shows resistance to beta-lactams through the aforementioned mechanisms (inactivation via hydrolysis, increased efflux, reduced influx, and protection against the ATB). The different types of mechanisms of resistance to beta-lactams are shown in Table 1.3
A. baumannii. Mechanisms of resistance to beta-lactams.
B- lactamase | Variants | Resistance profile |
---|---|---|
Class A | Broad spectrum beta-lactamases: TEM-1, TEM-2 | Penicillins |
CARB-5, VEB-1, PER-1, PER-2, TEM-92, TEM-116. SHV-5, SHV-12, CTX-M-2, CTX-M-43 | Extended spectrum cephalosporins, aztreonam | |
Carbapenemases: KPC | Carbapenems, penicillins, cephalosporins, aztreonam | |
Class B | Carbapenemases: IMP, VIM, SPM, SIM and NDM | Carbapenems, penicillins, cephalosporins, aztreonam is not hydrolyzed |
Class D | Carbapenemases: OXA-23, OXA-24, OXA-58, OXA-51 | Carbapenems, penicillins, cephalosporins, weaker for third and fourth generation cephalosporins |
See Reference 3 for further details.
Resistance to polymyxins B and E (colistin): Acinetobacter baumannii has an external membrane that is semipermeable to the influx of essential elements and for the clearance of toxic components. Lipopolysaccharides (LPS) are located in the external surface and contain hydrophobic and negatively charged lipid A that interacts with the non-ribosomal lipopeptide cations of the polymyxins. This interaction results in destabilization of the external membrane, the uptake of polymyxins in the periplasmic space, and an increase in permeability secondary to alteration of the integrity of both the internal and the external part of the membrane. Although the precise mechanism of action is not known, the hydrophobic part of the polymyxins appears to be crucial for the induction of membrane damage, and in this regard a detergent-like effect has been suggested. The mechanisms of resistance to the polymyxins include: (a) alteration of the ATB through modification of lipid A of the LPS secondary to genetic mutations; (b) mutations of genes that are essential for the biosynthesis of lipid A and are associated to lipid A deficiency; (c) expressions associated with defects in the permeability and osmotic resistance of the external membrane, inducing an important elevation of the minimum inhibitory concentration (MIC) of the polymyxins; (d) insufficient concentration of constitutive cofactors for the formation of LPS that are essential for sensitivity to polymyxins; and (e) efflux pumps.4
Resistance to aminoglycosides (AGs): This is an example of acquired resistance, and involves three different mechanisms: (1) enzyme activity that modifies the AGs, weakening their binding capacity; (2) alteration of the target site of methyltransferase 16S rRNA; and (3) limitation of AG uptake due to loss of permeability or reactivation of the efflux pumps.5
Resistance to sulbactam: The mechanism underlying resistance to sulbactam is not fully clear, though it fundamentally appears to involve the mutation of penicillin-binding protein (PBP); the production of beta-lactamases can also contribute to the generation of resistance.5,6
Clinical infections due to A. baumanniiA. baumannii pneumoniaHospital-acquired (or nosocomial) pneumonia (HAP) and ventilator-associated pneumonia (VAP) due to AB are frequent (incidence 40–95%), and are associated to a poor patient prognosis, increased hospitalization and costs, and an overall mortality rate of 45–85%.6 Unfortunately, AB is highly resistant to most of the usually available ATB, and only a few drugs such as colistin, tigecycline and sulbactam may prove effective. The carbapenems, often in combination with other ATB, are fundamental elements in the treatment of serious infections. However, in recent years there has been an increase in the presence of AB strains resistant to carbapenems, and particularly to meropenem.7,8
Acinetobacter baumannii has a notorious capacity to form biofilms in the endotracheal tube, where it is very difficult for ATB to penetrate – thereby facilitating slow growth of the bacterium and increasing the risk of VAP. Bacterial colonization of the endotracheal tube or ventilator-associated tracheobronchitis (VAT) is a common phenomenon, characterized by increased purulent secretion and inflammation of the lower airway.9 The clinical presentation and diagnostic criteria of VAT and VAP may be quite similar, though a diagnosis of VAP requires confirmation of the presence of new or persistent infiltrates on the chest X-rays. This similarity and the sometimes difficult differential diagnosis (since infiltrates of extrapulmonary origin may be mistaken for VAP) have led some authors to postulate that VAT could represent a stage prior to the development of VAP and should be treated with ATB. However, there is controversy as to whether or not patients with VAT should be prescribed with ATB, in view of the risk of prolonging or increasing the infection due to multidrug resistant organisms. Furthermore, the impact which such treatment may have upon the course of the patient is not clear.10,11 The management of VAT with nebulized ATB is seen to be a better option, since nebulized formulations (particularly of colistin and aminoglycosides) reach important concentrations at lung level (up to 100 times the MIC of AB), with almost no spread to the plasma compartment – thus minimizing the adverse events associated with these drugs. Although the level of evidence is still low, nebulized ATB have achieved bacterial eradication and clinical improvement, with no impact upon mortality.12,13
Bacteremia due to A. baumanniiDifferent series have reported an incidence of bacteremia due to AB of between 36–64%, with a crude mortality rate of 34.0–43.4%, and with an attributable mortality rate that can reach 58.6%.14 One of the risk factors for bacteremia is the widespread use of invasive devices or procedures in these patients, such as orotracheal intubation and mechanical ventilation (MV), central venous catheters (CVCs), arterial catheters, bladder catheters (BCs), nasogastric tubes (NGTs), chest or abdominal drains, etc. In a meta-analysis, Zhou et al. established the following odds ratios (ORs) for bacteremia due to AB: MV: OR 4.79 (95% confidence interval [95%CI] 3.09–7.43); CVC: OR 6.25 (95%CI 2.58–15.11); BC: OR 2.55 (95%CI 1.49–4.36); and NGT: OR 4.70 (95%CI 2.79–7.91).15 Thus, one of the most important strategies for reducing bacteremia would be to minimize the duration of invasive procedures as far as possible.
The start of early and appropriate ATB treatment significantly reduces mortality due to AB, particularly in more seriously ill patients.14
Antibiotic treatment of severe infections due to A. baumanniiAntimicrobials of choiceInappropriate antimicrobial treatment is clearly associated to increased mortality in patients with severe infections due to A. baumannii (AB). In the case of AB with high resistance to carbapenems, the latter would not appear to be the best choice within an antimicrobial treatment strategy.7,16
PolymyxinsThe polymyxins are the antimicrobials with the greatest in vitro activity against A. baumannii. There are two types of polymyxins: polymyxin B and polymyxin E (colistin), with similar structures and antibacterial activity in vitro, though with different pharmacological properties. According to the Clinical and Laboratory Standards Institute (CLSI) and the European Committee on Antimicrobial Susceptibility Testing (EUCAST), the minimum inhibitory concentration (MIC) breakpoint for assuming antibacterial sensitivity is similar for both polymyxins: ≤2 mg/l.17–19 The mechanism of action of the polymyxins in part would involve disruption or rupture of the external and internal membranes of the bacterium through a self-uptake mechanism, initially with binding of the positively charged polymyxins to the negative charge of the lipopolysaccharides (LPS) in the external membrane of the bacterium via electrostatic and hydrophobic interactions.
Polymyxin E or colistin: At present, polymyxin E or colistin is the polymyxin most commonly used for the treatment of infections due to AB, and is the polymyxin most widely studied to date. The CLSI and the EUCAST18,19 establish the same MIC breakpoint for sensitivity but different breakpoints for resistance (Table 2).
According to the International Consensus Guidelines for the Optimal Use of the polymyxins,20 it is advisable to use polymyxin B, except in the case of urinary tract infections (UTIs). The drug is administered as colistin sodium methanesulfonate (CSM), an inactive prodrug that is hydrolyzed to colistin base (its active form) in the tissues.
Seventy percent of the administered CSM is excreted through the renal tubules, with only 30% being hydrolyzed to colistin base. In this process, colistin base also undergoes renal and extrarenal excretion while reaching the infected tissues.17 An important confounding factor for the effective use of CSM is the difference in terminology regarding the administration dose, expressed in international units (IUs) or in mg, depending on the country. In general, one million IUs of CSM is equivalent to 80 mg of CSM or 34 mg of colistin base. Following the intravenous administration of CSM, colistin base is slowly formed in approximately 36–48 h, reaching the stable minimum bactericidal concentration (MBC) in plasma of 2 mg/l when administered every 8 h in patients with good renal function. This low initially formed concentration of colistin base has been a challenge for defining the optimum dose of CSM and its form of administration. In order to counter such slow formation of colistin, it is advisable to administer a loading dose of 9–10 million UI or 4–5 mg/kg, and then to continue with a maintenance dose of 9–10 million UI/day or 4–5 mg/kg/day, at 12-h intervals. The loading dose is independent of renal function. The maintenance doses in turn are to be adjusted to renal function in order to keep the MBC between 2 and 3 mg/l. In situations of hyperfiltration (glomerular filtration rate [GFR] >130 ml/min) and in patients subjected to renal replacement therapy, colistin base may be filtered in excess, thereby falling short of the suggested MBC, and requiring doses equal to those administered in patients with normal renal function. Furthermore, due consideration is required of the intra-individual variability of renal function, which may improve or worsen during the treatment, thereby requiring dose modifications.16,17,20
Tables 3 and 4 summarize the recommendations of the International Consensus Guidelines for the Optimal Use of the polymyxins.20
Colistin sodium methanesulfonate (CSM) dosing according to creatinine clearance for a plasma colistin concentration of 2 mg/l.
Loading dose, independent of creatinine clearance: 4–5 mg/kg or 9–10 million IU | ||
---|---|---|
Cl, creatinine ml/min | CBA, mg/day | Million IU/day |
0 | 130 | 3.95 |
5 to <10 | 145 | 4.40 |
10 to <20 | 160 | 4.85 |
20 to <30 | 175 | 5.30 |
30 to <40 | 195 | 5.90 |
40 to <50 | 220 | 6.65 |
50 to <60 | 245 | 7.40 |
60 to <70 | 275 | 8.35 |
70 to <80 | 300 | 9.00 |
80 to <90 | 340 | 10.3 |
≥ 90 | 360 | 10.9 |
Cl: clearance; CSM: colistin sodium methanesulfonate; CBA: colistin base activity.
See Reference 20 for further details.
Additional dosing of colistin according to renal replacement therapy.
a.- Intermittent hemodialysis (IHD) (duration ≈ 3–4 h): |
* Day no IHD: 130 mg or 3.95 million IU |
* Day IHD: extra dose after IHD |
- IHD 3 h: 40 mg or 1.2 million IU |
- IHD 4 h: 50 mg or 1.6 million IU |
b.- Slow dialysis therapies (duration 8–10 h) (SLED: sustained low-efficiency dialysis): |
* Add 10% of the basal dose x every 1 h of dialysis: ≈ 260 mg/d (130 + 130) or 7.9 million IU/day, at 12-h intervals |
c.- Continuous renal replacement therapy (CRRT) (duration 24 h): |
* 440 mg/d or 13.3 million IU/day, at 12-h intervals |
See Reference 20 for further details.
Polymyxin B (PMB): The drug is administered directly in its active form. In different countries the dose can also be expressed in mg or in IU, considering 1 mg = 10,000 IU.
Compared with CSM, only a few small studies have evaluated the pharmacokinetics of PMB following its intravenous administration. Elimination of the drug is fundamentally extrarenal, with less than 1% of the administered dose being recovered as unmodified substance in urine. Renal function does not greatly affect the plasma concentration of PMB and should not be used to adjust the dose.21 In a way similar to colistin, PMB is removed during dialysis. However, dose adjustment in patients subjected to renal replacement therapy is not advised, due to the limited available clinical data.22
Most of the studies on the pharmacodynamics of the polymyxins have been carried out with colistin. The latter exhibits rapid concentration-dependent bactericidal activity against AB and P. aeruginosa, with a minimum post-antibiotic effect. However, the best parameter for describing its antimicrobial activity (PK/PD index) is the ratio between the free area under the concentration-time curve and MIC (fAUC/MIC).23 Despite the rapid initial bacterial mortality, regrowth can manifest very quickly (as soon as within 2 h after initial exposure).23–26 In patients with good renal function, the administration of PMB is very likely to yield higher plasma concentrations over MIC than CSM because: (a) renal function influences the distribution of CSM but not of PMB; and (b) the in vivo conversion to colistin is slow and incomplete.
The pharmacodynamic behavior of PMB is very similar to that of colistin, with a similar rapid bactericidal effect upon AB, K. pneumoniae and P. aeruginosa in vitro, followed by rapid regrowth.27–29 In polymyxin hetero-resistant strains, amplification of the subpopulations resistant to polymyxins has been shown to play an important role in the rapid emergency of resistance.30–32
Toxicity of the polymyxins: Nephrotoxicity is the most common and dose-limiting adverse effect of these drugs when administered via the intravenous route, with an incidence of up to 60%.33,34 Polymyxin B and colistin accumulate in the renal tubules, possibly through active uptake mechanisms mediated by megalin and PEPT2 transporters.35,36 Such extremely high concentrations in the renal tubular cells cause dramatic changes in mitochondrial morphology, loss of cytoplasmic membrane potential, apoptosis, and cell cycle arrest. The exact mechanism underling polymyxin uptake by the tubular cells and subsequent cell death remains unclear.37,38
Nebulized colistinA number of studies have examined the use of nebulized colistin versus the combination of nebulized colistin plus intravenous colistin versus intravenous colistin alone, in application to ventilator-associated pneumonia (VAP), with the observation of greater clinical improvement when using nebulized colistin versus the other two options. Nebulized colistin reaches high concentrations in alveolar fluid (up to 100 times the MIC of AB), resulting in bacterial death within a few fours and without penetration into the plasma compartment (low toxicity). These concentrations are impossible to reach through intravenous administration without exposing the patient to greater toxicity.39,40
TigecyclineTigecycline is a glycylcycline with activity against gram-negative bacilli and gram-positive cocci. However, it has only been approved by the United States Food and Drug Administration (FDA) for complicated infections of the abdominal cavity, infections of the skin and appendages, and for community-acquired pneumonia. It has no indication for bacteremic or urinary infections. The FDA published an alert on the increased mortality risk with tigecycline compared with other antibiotics for the treatment of similar serious infections. This increased mortality risk was more manifest in patients with severe hospital-acquired pneumonia (HAP) or with ventilator-associated pneumonia (VAP), and so the drug was not approved for the treatment of these pneumonias.41
The recommended standard dosage comprises a loading dose of 100 mg, followed by 50 mg every 12 h. Recently, some authors have suggested double dosing (a loading dose of 200 mg, followed by 100 mg every 12 h) in order to optimize the antibacterial effect and achieve clinical healing in obese patients, with HAP, and with VAP.42,43 In patients with severe pneumonia (HAP, VAP), these high doses were associated to an improved patient course, since the concentrations of tigecycline in pulmonary endothelial fluid are insufficient with the standard dosage, particularly in the case of infections due to AB with MIC >2 mg/l, usually in combination with another antibiotic. At these double doses, tigecycline was found to be well tolerated, with no relevant toxicity.43
SulbactamSulbactam is a beta-lactamase inhibitor with bactericidal action against a number of strains of AB resistant to other drugs. It is marketed in combination with a beta-lactam, e.g., as ampicillin-sulbactam or cefoperazone-sulbactam. Its most important action is irreversible binding to the enzymes produced by the pathogen, resulting in the inhibition of beta-lactamase activity against the beta-lactam. The antimicrobial property that distinguishes sulbactam from other beta-lactamase inhibitors is its activity against AB.
Sulbactam is considered to be an ATB with time-dependent antibacterial activity, i.e., the pharmacokinetic/pharmacodynamic (PK/PD) parameter that best correlates to its efficacy is the percentage during which the free drug remains more than 50% of the dosing interval above the MIC of AB. In order to achieve this efficacy, the best way to administer sulbactam is in the form of a prolonged four-hour infusion of each dose.44 Therefore, the suggested dosage, particularly for less sensitive isolates (MIC of up to 8 mg/l), is 3 g of sulbactam every 8 h in a prolonged four-hour infusion.44,16
CefiderocolCefiderocol is a siderophore cephalosporin with a peculiar mechanism for penetrating the external cell membrane of gram-negative pathogens. The drug uses the iron absorption system of the bacterium to gain access to the interior of the cell. Cefiderocol has potent activity against a broad range of enterobacteria and non-fermenting gram-negative bacilli such as Pseudomonas aeruginosa and Acinetobacter baumannii – including strains resistant to carbapenems. This new cephalosporin is not affected by type VIM or NDM metallobetalactamases or by class D carbapenemases (OXA-23 or OXA-51), which are the main enzyme mechanisms of AB for inactivating beta-lactams.45
The CREDIBLE study46 is an open-label phase 3 clinical trial on the efficacy and safety of cefiderocol or of the best available therapy (BAT) for the treatment of a broad range of serious infections caused by pathogens resistant to carbapenems, regardless of the infection site involved. Although the clinical and microbiological outcomes were globally similar for both cefiderocol and BAT, in the subgroup of patients with infections due to A. baumannii the mortality rate was higher in the group treated with cefiderocol than in the group that received BAT (50% versus 18%) (See Fig. 1).
In contrast, the APEKS-NP study is a double-blind clinical trial evaluating cefiderocol versus meropenem in patients with nosocomial pneumonia due to gram-negative bacilli.47 The results evidenced the non-inferiority of cefiderocol, and a sub-analysis of the patients with pneumonia due to AB revealed no differences between the two groups in terms of either mortality at 14 days (primary endpoint of the study) or the rest of the secondary endpoints (Fig. 1).
Based on the results of these studies, and mainly on the findings of the CREDIBLE trial, both the European guides (ESCMID and ESICM) and the American guides of the IDSA disadvise the use of cefiderocol in infections produced by AB, and if the drug must be prescribed due to a lack of other options, it should be administered whenever possible in combination with another antibiotic known to be active in vitro.48,49
More recently, a retrospective observational study has reported the experience with cefiderocol in the treatment of severe infections due to AB (79 patients with bacteremia, 35 with VAP, and 10 with other types of infections) in an Italian hospital.50 A comparison was made of 47 patients treated with cefiderocol (32% in monotherapy) versus 77 patients receiving treatment based on colistin (only 17% in monotherapy). The mortality rate at 30 days was significantly higher in the group that received colistin (55.8% versus 34%; p = 0.018), and the multivariate analysis identified the administration of treatment with cefiderocol as a protective factor against mortality. As expected, nephrotoxicity was seen to be more frequent in the colistin group.
Monotherapy or combination therapyThe clinical benefit of monotherapy versus combination therapy remains subject to debate because of the lack of firm evidence from adequate randomized controlled trials - most of the available studies being of an observational nature. López-Cortés et al.51 evaluated 101 patients with sepsis due to multidrug resistant AB in 28 Spanish hospitals: 67.3% of the patients received monotherapy while 32.7% received combination therapy. Pneumonia was the most frequent infection (50.5%), and of these cases, 68.6% corresponded to VAP. Colistin (67.6%) and the carbapenems (14.7%) were the most frequently used antibiotics in monotherapy, while the most common combination therapies consisted of colistin plus tigecycline (27.3%) and carbapenems plus tigecycline (12.1%). In the multivariate analysis, the survival rate at 30 days showed no differences between the two treatment schemes (monotherapy 23.5% and combination therapy 24.2%).
Table 5 summarizes the results of 6 randomized studies evaluating monotherapy (MT) versus combination therapy (TC) in infections due to multidrug resistant AB.52–57 Some authors documented a 20% incidence of antagonism in vitro between the studied antibiotics, such as colistin, tigecycline, meropenem, sulbactam or aminoglycosides.58
Monotherapy versus combination therapy. Randomized trials.
Study, year (Ref.) | Population | Treatment | Results |
---|---|---|---|
Paul M, et al., 201852 | 406 patients with pneumonia or bacteremia due to GNB resistant to carbapenems; 77% were due to AB | Colistin (MT) vs colistin plus meropenem (CT) | MT vs CT: no significant difference in clinical failure and mortality at 14 days: 79% vs 73%. Similar results among patients with infection due to AB |
Durante-Mangoni E, et al., 201353 | 210 patients with infections due to AB | Colistin (MT) vs colistin plus rifampicin (CT) | MT vs CT: no difference in mortality at 30 days (42.9% vs 43.3%). Significant difference in microbiological eradication in CT (p = 0.034) |
Aydemir H, et al., 201354 | 43 patients with VAP due to AB | Colistin (MT) vs colistin plus rifampicin (CT) | MT vs CT: no difference in mortality: 63.6% vs 38.1%; (p = 0.017). Difference in time to microbiological eradication: 4.5 ± 1.7 days vs 3.1 ± 0.5 days (p = 0.029) |
Park HJ, et al., 201955 | 9 patients with pneumonia due to AB | Colistin (MT) vs colistin plus rifampicin (CT) | MT vs CT: no difference in clinical healing (80% vs 66.7%; p > 0.99) or microbiological response (40% vs 100%; p = 0.19) |
Makris D, et al., 201856 | 39 patients with VAP due to AB | Colistin (MT) vs colistin plus ampicillin/sulbactam (CT) | MT vs CT: difference in clinical healing (15.8% vs 70%; p = 0.001). No difference in adjusted mortality at 28 days (63.2% vs 50%; p = 0.32) |
Sirijatuphat R, et al., 201457 | 94 patients with infection due to AB; pneumonia in 78% of MT and in 74% of CT | Colistin (MT) vs colistin plus fosfomycin (CT) | MT vs CT: no difference in mortality at 28 days (53.8% vs 44.2%; p = 0.50) or clinical response (56.4% vs 62.8%; p = 0.65). Significant difference in bacterial eradication (84.5% vs 100%; p = 0.023) |
GNB: gram-negative bacilli; MT: monotherapy; CT: combination therapy; AB: A. baumannii; VAP: ventilator-associated pneumonia.
As seen in these studies, ATB treatment of multidrug resistant AB is a great challenge, particularly in serious infections. There is no clear ATB standard of care regimen with which to assess the effectiveness of the different treatment regimens. Those AB strains showing resistance to carbapenems generally may have already acquired resistance to most of the antibiotics that were considered to be active (sulbactam, aminoglycosides, fluoroquinolones).59
The recent IDSA guides59 suggest that ATB treatment with a single drug substance could suffice in the case of mild infections caused by AB resistant to carbapenems (AB-RCB) - preferably ampicillin/sulbactam. In contrast, the guides recommend combination therapy in moderate to severe infections involving at least two antibiotics with in vitro activity – the clinical evidence being moderate, at least until an adequate clinical response is confirmed.
The latest ESICMID guides60 provide the following recommendations regarding ATB treatment for infections caused by AB resistant to carbapenems (AB-RCB):
a.- Combination therapy with polymyxins plus meropenem is not advised (strong recommendation against use, high level of evidence), in the same way as the combination of polymyxin plus rifampicin (strong recommendation against use, moderate level of evidence)
b.- In the case of severe infections with a high risk of AB-RCB, the guides recommend combination therapy including two antibiotics with in vitro activity among the available options (polymyxins, aminoglycosides, tigecycline, sulbactam) (conditional recommendation of use, very low level of evidence)
c.- In the case of infections due to AB-RCB with MIC for meropenem ≤8 mg/l, the recommendation is to prescribe combination therapy with carbapenems, using high doses as a prolonged or extended infusion (good practice recommendation, expert opinion)
d.- In the case of patients with hospital-acquired pneumonia and VAP due to AB-RCB sensitive to sulbactam, ampicillin/sulbactam (conditional recommendation, low level of evidence) is recommended
e.- In the case of patients with AB-RCB resistant to sulbactam, the recommendation is to prescribe a polymyxin or high doses of tigecycline if they exhibit in vitro activity (lack of evidence for recommending a preferred ATB)
f.- Treatment with cefiderocol for infection due to AB-RCB is not advised (conditional recommendation against use, low level of evidence)
Two meta-analyses have evaluated the data obtained from observational studies and randomized clinical trials involving different treatments for infections caused by AB-RCB.61,62 In a meta-analysis published in 2021, Liu et al.61 included 18 studies that totaled 1835 patients. The authors found ampicillin/sulbactam at a maximum daily dose of 18 g in combination with a second drug to be the most effective option for reducing mortality. Nephrotoxicity was less manifest in the regimens with ampicillin/sulbactam than in the regimens based on polymyxins. Jung et al.62 in turn published a meta-analysis in 2017 comprising 23 observational studies or randomized clinical trials with a total of 2118 patients. These authors found sulbactam to be the drug with the greatest impact in terms of the reduction of mortality on evaluating regimens based on sulbactam versus regimens based on polymyxins or tetracyclines. Unfortunately, no comparison of adverse events between the different treatment schemes could be made.
Duration of antibiotic treatmentAlthough a number of guides recommend shortening the duration of ATB treatment due to the worrisome increase in antimicrobial resistances, this advice is not predominantly followed in the real-life scenario, particularly in patients with serious infections and who are often in critical condition, with shock and multiorgan dysfunction.63
In recent years, attention has focused on disruption of the intestinal microbiome, which can lead to colonization by pathogenic organisms, and on how this situation may constitute an antimicrobial resistance reservoir, e.g., characterized by resistance to beta-lactams or plasmid-mediated quinolone resistance.64
A recent meta-analysis of 19 randomized studies including 2867 patients examined the results of short versus prolonged ATB treatment. No significant differences were observed in terms of the microbiological healing rate, recurrence of infection or mortality at 45, 60 and 180 days. The heterogeneity between studies was not significant in relation to these different results. The authors concluded that a short ATB course may be safe for hospitalized patients with frequent infections such as pneumonia, urinary tract infections or intraabdominal infections, and that clinical and microbiological resolution can be achieved with no negative impact upon mortality or recurrence.65
The optimum duration of ATB treatment must balance efficacy or bacterial killing against the adverse effects of the medication, such as toxicity or the development of resistance. Infections that are frequent in immunocompetent critical patients could be treated with a short course of 7 days or less, provided use is made of an active or appropriate ATB and at the adequate doses, with control of the infectious focus where applicable. The integration of different parameters such as the clinical signs of resolution of the infection, biomarkers and clinical judgment may help define the optimum duration of ATB treatment in serious infections caused by multidrug resistant organisms or extremely resistant pathogens that prove life-threatening for the patient.66 In the case of patients with confirmed infection, the duration of ATB treatment may be determined by the interaction among the host factors, pathogen characteristics, and ATB features, and by other pre-requirements for defining the shortening of therapy, such as (i) improvement of the clinical signs and symptoms; (ii) adequate and timely control of the infectious focus; (iii) confirmed sensitivity to the administered ATB when the bacteriological report is received (appropriate empirical treatment); and (iv) adequate monitoring of the pharmacokinetics/pharmacodynamics (PK/PD) of the indicated antibiotics.66
The duration of ATB therapy for infections caused by AB has not been clearly established. Some studies consider a short course of treatment to be less than 10 days, while a long course is taken to represent 10 or more days.67,68 However, other studies define a long course as corresponding to 7 or 8 days of treatment.69
In a now classical study, Chastre et al.70 compared an ATB course of 8 days (n = 197) versus 15 days (n = 204) in patients with VAP. The results showed: (i) an equally favorable course and duration of stay in the Intensive Care Unit (ICU); (ii) equal mortality (18.8% vs 17.2%); and (iii) equal recurrence (28.9% vs 26%), relapse (16.8% vs 11.3%), and superinfection rates (19.8% vs 18.6%). On analyzing the subgroup of patients with infection due to P. aeruginosa and A. baumannii, no differences were recorded in terms of ICU stay, multiorgan failure or mortality. However, the recurrence rate was significantly higher in the group of 8 days of treatment versus the group of 15 days of treatment (40.6% vs 25.4%; p = 0.003) – though recurrence due to multidrug resistant organisms was significantly lower in the group of 8 days of treatment (42.1% vs 62.0%; p = 0.04). The authors concluded that a short treatment course of 8 days is feasible in patients with VAP, even in the context of infections caused by non-fermenting gram-negative bacilli.
According to the clinical practice guides of the IDSA/ATS, a short course of 7 days instead of a longer course is strongly advised in hospital-acquired pneumonia and in VAP, even in infections caused by non-fermenting gram-negative bacilli such as P. aeruginosa and A. baumannii.71
In their review, Busch et al.72 defined the following ATB treatment courses according to the site of the infection:
a.- bacteremia: 10 to 14 days
b.- ventilator-associated pneumonia (VAP): 7 to 10 days
c.- hospital-acquired pneumonia: 7 to 10 days
d.- community-acquired pneumonia: 5 to 7 days
e.- complicated intraabdominal infection: 5 to 7 days
f.- complicated urinary infection: 5 to 7 days
Lastly, the guidelines of the Surviving Sepsis Campaign 202173 referred to adults with sepsis or septic shock and adequate control of the infectious focus suggest the following preferences in relation to ATB treatment:
- (i)
Short rather than long ATB course (weak recommendation, very low level of evidence)
- (ii)
De-escalation of ATB treatment versus continuation with fixed duration (weak recommendation, very low level of evidence)
- (iii)
Use of procalcitonin plus clinical assessment versus clinical assessment alone in deciding when to suspend treatment (weak recommendation, low level of evidence)
The risk associated with a long ATB treatment course was evaluated by Curran et al.74 in a meta-analysis of 71 randomized controlled studies (23,174 patients). Every day of ATB therapy was associated to a 4% increase in the odds of experiencing an adverse event (OR 1.04, 95%CI: 1.02–1.07). The daily odds of severe adverse events also increased (OR 1.09, 95%CI: 1.00–1.19). The daily increase in the odds of superinfection and of resistance to ATB was OR 0.98 (0.92–1.06) and OR 1.03 (0.98–1.07), respectively.
These data can allow balancing of the risks versus the benefits when a prolonged treatment course is decided.
Antibiotics under developmentAlthough the list could be longer, in this section we review two new molecules with activity against AB - though clinical data are still lacking, particularly in relation to the second drug.
Eravacycline: This drug is a tetracycline; it therefore inhibits bacterial protein synthesis by binding to the 30S ribosomal subunit. Eravacycline possesses strong broad spectrum activity against gram-negative bacilli (except P. aeruginosa and Burkholderia spp.) and gram-positive cocci, including anaerobes, as well as against atypical bacterial pathogens. Among the gram-negative bacilli, it is active against carbapenemase-producing enterobacteria (including carbapenemases KPC, VIM, IMP, NDM and OXA-48), as well as against AB resistant to carbapenems. The drug is 4-8 times more potent than tigecycline against AB.75 Very little clinical experience with this antibiotic is available in infections due to AB. Indeed, although eravacycline could be a valid option, a recent retrospective study of patients with severe infections caused by AB does not report very favorable results, particularly in patients with bacteremia, in which the mortality rate was seen to be higher with eravacycline versus the best available therapy.76
Durlobactam: This drug is a novel broad spectrum serine type beta-lactamase (Ambler classes A, C and D) inhibitor that moreover possesses weak intrinsic activity against AB. Durlobactam improves the activity of various beta-lactams against AB, though the most potent combination corresponds to administration with another beta-lactamase inhibitor: sulbactam. In a recent study, the in vitro activity of the combination durlobactam/sulbactam was evaluated in application to 1722 clinical isolates of AB, collected on 5 continents in 2016 and 2017. Over 50% of these samples proved resistant to carbapenems. Durlobactam/sulbactam presented an MIC50 value of 1 g/ml, versus 8 g/ml in the case of sulbactam, with an MIC90 of 64 g/ml. In this study, colistin was the only traditional antibacterial with activity similar to that of durlobactam/sulbactam.77 At present there is a lack of clinical information warranting the usefulness of this combination of beta-lactamase inhibitors in infections caused by AB. A phase 3 clinical trial (the ATTACK study) has been completed, comparing durlobactam/sulbactam versus colistin in severe infections due to AB. The results have not yet been published, though a preliminary report affirms that the non-inferiority criterion corresponding to the primary endpoint (crude mortality at 28 days) has been met.78
ConclusionsAcinetobacter baumannii is a non-fermenting gram-negative bacillus with great multidrug resistance that causes a range of serious nosocomial infections associated to high morbidity-mortality particularly in critical patients, with septic shock and multiorgan failure. In recent decades, A. baumannii has shown increased resistance to multiple antibiotics - including carbapenems - through multiple resistance mechanisms that represent a complication and an enormous challenge in deciding effective ATB treatment and its duration. The understanding of these mechanisms and of the risk factors for developing AB infections is crucial in order to reduce their incidence in ICUs and in hospitals, with the minimization of bacterial colonization and prevention of the transmission A. baumannii infections.