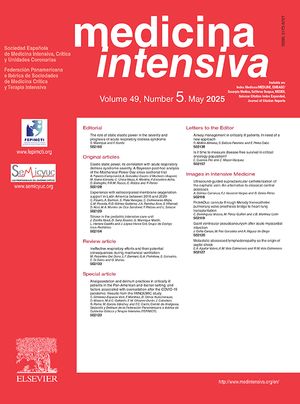
Edited by: Rosario Amaya Villar - Unidad de Cuidados Intensivos, Hospital Universitario Virgen del Rocio, Sevilla, España
Last update: January 2025
More infoWe examined weather a protocol for fraction of inspired oxygen (FiO2) adjustment can reduce hyperoxemia and excess oxygen use in COVID-19 patients mechanically ventilated.
DesignProspective cohort study.
SettingTwo intensive care units (ICUs) dedicated to COVID-19 patients in Brazil.
PatientsConsecutive patients with COVID-19 mechanically ventilated.
InterventionsOne ICU followed a FiO2 adjustment protocol based on SpO2 (conservative-oxygen ICU) and the other, which did not follow the protocol, constituted the control ICU.
Main variables of interestPrevalence of hyperoxemia (PaO2>100mmHg) on day 1, sustained hyperoxemia (present on days 1 and 2), and excess oxygen use (FiO2>0.6 in patients with hyperoxemia) were compared between the two ICUs.
ResultsEighty two patients from the conservative-oxygen ICU and 145 from the control ICU were included. The conservative-oxygen ICU presented lower prevalence of hyperoxemia on day 1 (40.2% vs. 75.9%, p<0.001) and of sustained hyperoxemia (12.2% vs. 49.6%, p<0.001). Excess oxygen use was less frequent in the conservative-oxygen ICU on day 1 (18.3% vs. 52.4%, p<0.001). Being admitted in the control ICU was independently associated with hyperoxemia and excess oxygen use. Multivariable analyses found no independent relationship between day 1 hyperoxemia, sustained hyperoxemia, or excess FiO2 use and adverse clinical outcomes.
ConclusionsFollowing FiO2 protocol was associated with lower hyperoxemia and less excess oxygen use. Although those results were not associated with better clinical outcomes, adopting FiO2 protocol may be useful in a scenario of depleted oxygen resources, as was seen during the COVID-19 pandemic.
Evaluar si un protocolo para el ajuste de la FiO2 reduce la hiperoxemia y el uso excesivo de oxígeno en pacientes con COVID-19 en ventilación mecánica.
DiseñoEstudio de cohorte prospectivo.
ÁmbitoUnidades de cuidados intensivos (UCI) dedicadas a pacientes con COVID-19 en Brasil.
PacientesPacientes con COVID-19.
IntervencionesUna UCI siguió un protocolo de ajuste de FiO2 basado en SpO2 (UCI de oxigenoterapia conservadora, N=82) y la otra no siguió el protocolo (UCI control, N=145).
Principales variables de interésPrevalencia de hiperoxemia (PaO2>100mmHg) en el día 1, hiperoxemia sostenida (presente en los días 1 y 2) y exceso de uso de oxígeno (FiO2>0,6 en pacientes con hiperoxemia) entre las 2 UCI.
ResultadosLa UCI de oxigenoterapia conservadora presentó menor prevalencia de hiperoxemia en el día 1 (40,2 vs. 75,9%; p<0,001) y de hiperoxemia sostenida (12,2 vs. 49,6%; p<0,001). El uso excesivo de oxígeno fue menos frecuente en la UCI de oxigenoterapia conservadora el día 1 (18,3 vs. 52,4%; p<0,001). El ingreso en la UCI control se asoció de forma independiente con la hiperoxemia y el uso excesivo de oxígeno. Los análisis multivariables no encontraron una relación independiente entre hiperoxemia o uso excesivo de FiO2 y resultados clínicos adversos.
ConclusionesSeguir el protocolo de FiO2 se asoció con menor hiperoxemia y menor consumo de oxígeno en exceso. Aunque esos resultados no se asociaron con mejores resultados clínicos, la adopción del protocolo FiO2 puede ser útil en un escenario de recursos de oxígeno agotados, como se vio durante la pandemia de COVID-19.
Patients with severe COVID-19 pneumonia may require invasive mechanical ventilation (MV) due to acute respiratory distress syndrome (ARDS). MV is highly effective in increasing oxygenation and reverting hypoxemia, even in patients with severe forms of ARDS.1,2 However, during MV, patients are frequently exposed to high fractions of inspired oxygen (FiO2), sometimes higher than necessary.3,4 This exposure to high FiO2 can induce pulmonary inflammation due to excessive production of reactive oxygen species, as demonstrated by experimental studies.5,6 Another possible consequence of ventilating patients with high FiO2 is the occurrence of hyperoxemia, defined by arterial partial pressure of oxygen (PaO2) higher than 100mmHg.7 Hyperoxemia has been associated with worse outcomes in critically ill patients with acute brain or myocardial injury, and patients resuscitated post cardiac arrest, possible due to vasoconstriction in the cerebral and coronary circulation.8
However, the impact of hyperoxemia in mechanically ventilated patients due to ARDS is still controversial. The LUNG SAFE study showed that, among 2005 ARDS patients, 30% had hyperoxemia on the first day of MV and 12% had hyperoxemia on both the first and second days of MV. Despite its frequency, hyperoxemia was not associated with higher mortality in this observational study.7 Two recent randomized clinical trials compared a conservative oxygen therapy (targeting a PaO2 between 55 and 70mmHg) with a liberal oxygen therapy (targeting a PaO2 between 90 and 105mmHg), in patients with ARDS.9,10 Those studies did not show significant differences between the two groups in mortality, length of intensive care unit (ICU) stay or duration of MV. Those results showed that a more conservative oxygen use is safe and can reduce costs and spare oxygen, a gas that became scarce in some regions during the COVID-19 pandemic.11
During the COVID-19 pandemic, several ICUs were overburdened and had to treat a high number of patients, several times with insufficient expert staff and lack of equipment. The overload ICUs and the overwhelmed staffs might have reduced the compliance with the best practices in MV and were associated with excess mortality.12 Therefore, our hypotheses are: 1. hyperoxemia and excess oxygen use are frequent among COVID-19 patients under MV; 2. following a structured protocol to reduce FiO2 based on the peripheral oxygen saturation (SpO2) reduces the occurrence of hyperoxemia and excess oxygen use. To investigate those hypotheses, we compared two cohorts of COVID-19 patients, one conducted in an ICU with a protocol to adjust the FiO2 systematically and the other without a protocol to adjust the FiO2.
Patients and methodsThis is a prospective cohort study conducted in two ICUs dedicated to COVID-19 patients in two public hospitals in Juiz de Fora (Minas Gerais, Brazil): Federal University of Juiz de Fora University Hospital and Regional Doutor João Penido Hospital. Patients were included from March, 2020 to June, 2021. The study protocol followed the ethical principles of the Declaration of Helsinki and was approved by the Ethics Committees of both hospitals and written informed consent was obtained from the patients’ next of kin (protocol number 3.949.165).
PatientsConsecutive patients aged 18 years or older, admitted to one of the two participating ICUs with COVID-19 confirmed by RT-PCR, and who received invasive MV were eligible for participating in the study. We excluded patients who had received invasive MV for more than 24h before admission in the participating ICUs, patients who were ventilated for less than 48h, and those for whom life-sustaining treatment was withheld. Since the study focus was on hyperoxemia and excess oxygen use, we excluded patients with hypoxemia (PaO2<55mmHg, regardless of the FiO2) on day 0, defined as the calendar day when the patient was intubated.
FiO2 adjustment protocolA FiO2 adjustment protocol was already applied in one of the participant ICUs. The same protocol was also applied in the ICU dedicated to COVID-19 patients in this hospital (conservative-oxygen ICU). According to this protocol, patients under MV and with positive end-expiratory pressure (PEEP) equal or lower than 8cmH2O were evaluated every 1h by a nurse, who adjusted FiO2 based on SpO2. If SpO2 was higher than 96%, FiO2 was reduced in 10% (absolute value); if SpO2 was between 93% and 96%, FiO2 was maintained; and if SpO2 was lower than 93%, the doctor in charge was called. In patients with PEEP higher than 8cmH2O, the nurses did not adjust FiO2, but they called the doctor in charge if SpO2 was lower than 93% (eFigure 1 in Supplementary Appendix). In the other hospital, the ICU dedicated to COVID-19 patients did not adopt any FiO2 protocol and constituted the control-ICU.
The other ventilatory parameters were set by the doctors in charge, who were orientated to keep a protective MV (Appendix A). Neither inhaled nitric oxide (NO), nor extracorporeal membrane oxygenation (ECMO) were available in the ICUs.
Data collectionAt ICU admission, the following patient's characteristics were prospectively recorded: age, sex, body-mass index (BMI), Charlson Comorbidity Index, Simplified Acute Physiology Score (SAPS-III), Sequential Organ Failure Assessment (SOFA), and laboratory tests.
The following ventilatory parameters were collected on day 1 and day 2, as close as possible to 8a.m. each day: tidal volume (VT), respiratory rate, FiO2, PEEP, plateau pressure, driving pressure (plateau pressure minus total PEEP), respiratory system compliance (VT divided by driving pressure). Arterial blood gas analysis was recorded simultaneously with the ventilatory parameters. For each day until ICU discharge or death, assessment was made as to whether patients were under MV or not. Patient survival was evaluated at day 90.
OutcomesThe primary outcomes were the prevalence of hyperoxemia and excess oxygen use on day 1 and day 2 in both cohorts. We defined hyperoxemia as PaO2>100mmHg and excess oxygen use as FiO2>0.6 in patients with hyperoxemia. Sustained hyperoxemia was defined as the presence of hyperoxemia on days 1 and 2.
Secondary outcomes included: occurrence of hypoxemia (defined as PaO2<55mmHg, regardless of FiO2), number of ventilator-free days at day 28, length of stay in the ICU, ICU mortality, hospital mortality, and 90-day mortality. Ventilator-free days were defined as calendar days of unassisted breathing for at least 24 consecutive hours. In patients who died by day 28, ventilator-free days were considered 0.
Statistical analysisA convenience sample was considered for this study, and consecutive patients were included. No assumptions were made for missing data. Categorical variables were expressed as absolute numbers and percentages and continuous variables, as medians and interquartile ranges. For categorical variables, the two cohorts were compared by the chi-square test; for continuous variables, they were compared by the Wilcoxon rank-sum test.
Multivariable logistic regression models considering the ICU admission (conservative-oxygen or control ICU) as the predictor of interest was constructed to assess variables independently associated with hyperoxemia and with excess oxygen use. The following variables were selected for initial assessment according to clinical relevance: age, gender, Charlson comorbidity index, SAPS-III, non-respiratory SOFA on day 1, laboratory tests at admission (D-dimer, C-reactive protein, ferritin, and lactic dehydrogenase), respiratory parameters on day 1 (respiratory system compliance, plateau pressure, driving pressure, PEEP, VT, PaO2/FiO2, PaCO2, pH, bicarbonate). Variables with a p<0.20 in the univariable prediction model were included in the multivariable model. Results were reported as odds ratio (OR) with 95% confidence interval (CI).
Another multivariable logistic regression model considering hyperoxemia on day 1, sustained hyperoxemia or excess oxygen use as the predictor of interest was constructed to assess variables independently associated with hospital mortality. The same variables selected above were initially assessed and those with a p<0.20 in the univariable prediction model were included in the multivariable model. Results were reported as OR with 95% CI.
All statistics tests were two-tailed with a significance level of 0.05. Data were analyzed with Stata 15.1 (StataCorp LP, College Station, TX, USA).
ResultsDuring the enrollment period, 82 from the conservative-oxygen ICU and 145 from the control ICU (Fig. 1). Table 1 shows demographic, clinical characteristics, and the ventilator settings on day 1 of MV from patients in the two participating ICUs.
Characteristics of the patients on day 1.
Conservative-oxygen ICU | Control ICU | p-Value | |
---|---|---|---|
Age (years), median (IQR) | 61 (47–70) | 65 (55–75) | 0.02 |
Male, n (%) | 44 (53.7) | 77 (53.1) | 0.93 |
SAPS III, median (IQR)a | 43 (39–52) | 46 (41–54) | 0.14 |
SOFA score, median (IQR) | 7 (5–8) | 6 (3–7) | <0.0001 |
Non-respiratory SOFA, median (IQR) | 4 (3–5) | 3 (1–5) | 0.06 |
Charlson index, median (IQR) | 2 (1–4) | 3 (2–5) | <0.001 |
PaO2/FiO2, median (IQR) | 189 (164–241) | 204 (145–260) | 0.87 |
ARDS severity, n (%)a | |||
Mild | 22 (29.7) | 29 (21.2) | 0.26 |
Moderate | 41 (55.4) | 78 (56.9) | |
Severe | 11 (14.9) | 30 (21.9) | |
PaCO2(mmHg), median (IQR) | 47 (40–54) | 45 (39–52) | 0.27 |
Arterial pH, median (IQR) | 7.33 (7.28–7.39) | 7.34 (7.27–7.41) | 0.87 |
Bicarbonate (mmol/L), median (IQR) | 25 (22–29) | 23 (21–27) | 0.02 |
C-reactive protein (mg/L), median (IQR) | 184 (85–275) | 142 (81–194) | <0.001 |
FiO2, median (IQR) | 0.5 (0.4–0.6) | 0.6 (0.5–0.8) | <0.0001 |
FiO2≥0.6, n (%) | 16 (19.5) | 72 (49.7) | <0.0001 |
Respiratory rate (breaths/min), median (IQR) | 25 (22–28) | 24 (20–26) | <0.001 |
Tidal volume (mL/kg PBW), median (IQR) | 6.5 (6.1–7.1) | 6.4 (5.9–7.4) | 0.58 |
Plateau pressure (cmH2O), median (IQR) | 22 (20–25) | 25 (22–28) | <0.0001 |
Driving pressure (cmH2O), median (IQR) | 12 (10–14) | 14 (12–16) | <0.001 |
PEEP (cmH2O), median (IQR) | 10 (8–12) | 12 (10–12) | <0.001 |
CRS(mL/cmH2O), median (IQR) | 33 (26–39) | 29 (24–35) | 0.03 |
Abbreviations: ARDS, acute respiratory distress syndrome; CRS, respiratory system compliance; FiO2, fraction of inspired oxygen; IQR, interquartile range; PaO2, arterial oxygen partial pressure; PaCO2, arterial carbon dioxide partial pressure; PBW, predicted body weight; PEEP, positive end-expiratory pressure; SAPSIII, simplified acute physiology score; SOFA, Sequential Organ Failure Assessment.
Patients from the conservative-oxygen ICU, compared with those from the control ICU, presented lower PaO2 and lower FiO2 on day 1 and day 2 of MV (Table 2). The PaO2 was lower in the conservative-oxygen ICU group in all different levels of FiO2 uses, on days 1 and 2 (Fig. 2a and b). The proportions of patients with hyperoxemia on day 1 and with sustained hyperoxemia were lower in the conservative-oxygen ICU (Table 2), a result that was consistent in all different levels of FiO2 uses (Fig. 2c and d). Density distributions of PaO2 on days 1 and 2 reveal different profiles between the two ICUs (Fig. 2e and f). Excess oxygen use was less frequent in the conservative-oxygen ICU on day 1 (18.3% versus 52.4%, p<0.001), and day 2 (10.9% versus 35.2%, p<0.001) (Table 2). No patients from both ICUs had hypoxemia on days 1 and 2 (Table 2).
Occurrence of hyperoxia, excess oxygen use and clinical outcomes in the two groups.
Conservative-oxygen ICU | Control ICU | p-Value | |
---|---|---|---|
PaO2at day 1 (mmHg), median (IQR) | 92 (81–112) | 125 (101–160) | <0.0001 |
PaO2at day 2 (mmHg), median (IQR) | 91 (78–106) | 121 (90–146) | <0.0001 |
Hyperoxemia at day 1, n (%) | 33 (40.2) | 110 (75.9) | <0.0001 |
Hyperoxemia at day 2, n (%) | 27 (32.9) | 95 (65.5) | <0.0001 |
Sustained hyperoxemia, n (%) | 10 (12.2) | 72 (49.6) | <0.0001 |
Hypoxemia at day 1, n (%) | 0 (0) | 0 (0) | 1 |
Hypoxemia at day 2, n (%) | 0 (0) | 0 (0) | 1 |
FiO2at day 1, median (IQR) | 0.50 (0.40–0.60) | 0.60 (0.50–0.80) | <0.0001 |
FiO2at day 2, median (IQR) | 0.47 (0.40–0.55) | 0.55 (0.50–0.70) | <0.0001 |
Excess oxygen use at day 1, n (%) | 15 (18.3) | 76 (52.4) | <0.0001 |
Excess oxygen use at day 2, n (%) | 9 (10.9) | 51 (35.2) | <0.0001 |
Ventilator free days (days), median (IQR) | |||
All | 0 (0–19) | 0 (0–16) | 0.46 |
Survivors at ICU discharge | 18 (7–22) | 16 (5–21) | 0.31 |
ICU length of stay (days), median (IQR) | |||
All | 16 (7–31) | 16 (9–27) | 0.91 |
Survivors at ICU discharge | 18 (11–33) | 16 (10–28) | 0.42 |
28-day mortality | 30 (36.6) | 55 (37.9) | 0.84 |
60-day mortality | 34 (41.6) | 74 (51.3) | 0.17 |
Hospital mortality | 40 (48.8) | 78 (53.8) | 0.46 |
Abbreviations: FiO2, fraction of inspired oxygen; ICU, intensive care unit; IQR, interquartile range; PaO2, arterial oxygen partial pressure.
Arterial oxygen tension and use of oxygen on days 1 and 2 of mechanical ventilation. A. Box plot of PaO2 at different ranges of FiO2 in the conservative-oxygen and control ICUs on day 1. B. Box plot of PaO2 at different ranges of FiO2 in the conservative-oxygen and control ICUs on day 2. C. Frequency of patients with hyperoxemia and normoxemia at different ranges of FiO2 in the conservative-oxygen and control ICUs on day 1. D. Frequency of patients with hyperoxemia and normoxemia at different ranges of FiO2 in the conservative-oxygen and control ICUs on day 2. E. Density distributions of PaO2 in the conservative-oxygen and control ICUs on day 1. F. Density distributions of PaO2 in the conservative-oxygen and control ICUs on day 2.
Multivariable analyses identified that being admitted in the control ICU and higher PaO2/FiO2 as factors independently associated with hyperoxemia on day 1, and being admitted in the control ICU, higher PaO2/FiO2, and lower protein C-reactive levels as factors independently associated with sustained hyperoxemia (Table 3). The independently factors associated with excess oxygen use on day 1 were being admitted in the control ICU and lower compliance of the respiratory system (Table 3).
Factors associated with day 1 hyperoxemia, sustained hyperoxemia and excess oxygen use.
Odds ratio (95% confidence interval) | p-Value | |
---|---|---|
Outcome – hyperoxemia at day 1 | ||
Being admitted to control ICU | 9.04 (3.94–20.73) | <0.0001 |
Non-respiratory SOFA | 1.00 (0.87–1.16) | 0.99 |
C-reactive protein admission (mg/L) | 1.00 (0.99–1.00) | 0.89 |
PaO2/FiO2 at day 1 | 1.02 (1.02–1.03) | <0.0001 |
Bicarbonate (mmol/L) | 0.96 (0.90–1.03) | 0.21 |
Driving pressure (cmH2O) | 1.08 (0.97–1.21) | 0.18 |
Outcome – sustained hyperoxemia (day 1 and 2) | ||
Being admitted to control ICU | 6.73 (2.98–15.19) | <0.0001 |
Non-respiratory SOFA score | 1.04 (0.92–1.18) | 0.53 |
C-reactive protein admission (mg/L) | 0.99 (0.99–1.00) | 0.04 |
PaO2/FiO2 at day 1 | 1.01 (1.01–1.01) | <0.0001 |
Bicarbonate (mmol/L) | 0.99 (0.93–1.05) | 0.67 |
Driving pressure (cmH2O) | 1.06 (0.96–1.17) | 0.23 |
Outcome – excess oxygen use at day 1 | ||
Being admitted to control ICU | 4.85 (2.44–9.61) | <0.0001 |
PEEP (cmH2O) | 1.18 (0.99–1.41) | 0.06 |
Plateau pressure (cmH2O) | 0.94 (0.83–1.06) | 0.29 |
CRS (mL/cmH2O) | 0.95 (0.91–0.99) | 0.02 |
Abbreviations: CRS, respiratory system compliance; FiO2, fraction of inspired oxygen; ICU, intensive care unit; PaO2, arterial oxygen partial pressure; PEEP, positive end-expiratory pressure; SOFA, Sequential Organ Failure Assessment.
Ventilator free days, length of stay in the ICU, 28-day mortality, 90-day mortality, and hospital mortality did not differ significantly between the two groups. Hyperoxemia on day 1, sustained hyperoxemia and excess oxygen use on day 1 were not independently associated with hospital mortality (Table 4).
Factors associated with hospital mortality in study population.
Factor | Odds ratio (95% CI), pHyperoxemia on day 1 as the predictor of interest | Odds ratio (95% CI), pSustained hyperoxemia as the predictor of interest | Odds ratio (95% CI), pExcess oxygen use on day 1 as the predictor of interest |
---|---|---|---|
Hyperoxemia on day 1 | 1.07 (0.48–2.39), p=0.86 | ||
Sustained hyperoxemia | 1.01 (0.47–2.14), p=0.98 | ||
Excess oxygen use on day 1 | 1.10 (0.54–2.24), p=0.80 | ||
Age | 1.08 (1.04–1.11), p<0.0001 | 1.08 (1.04–1.11), p<0.0001 | 1.08 (1.04–1.11), p<0.0001 |
SOFA score day 1 | 1.29 (1.10–1.52), p=0.002 | 1.29 (1.10–1.52), p=0.002 | 1.29 (1.10–1.52), p=0.002 |
Lactic dehydrogenase (IU/L) | 1.00 (0.99–1.00), p=0.33 | 1.00 (0.99–1.00), p=0.35 | 1.00 (0.99–1.00), p=0.33 |
Driving pressure (cmH2O) | 1.13 (1.02–1.26), p=0.02 | 1.14 (1.02–1.27), p=0.02 | 1.13 (1.02–1.26), p=0.02 |
Bicarbonate (mmol/L) | 1.04 (0.97–1.10), p=0.28 | 1.04 (0.97–1.10), p=0.29 | 1.04 (0.97–1.11), p=0.28 |
PaO2/FiO2 at day 1 | 0.99 (0.99–1.00), p=0.58 | 0.99 (0.99–1.00), p=0.60 | 0.99 (0.99–1.00), p=0.60 |
Renal replacement therapy | 2.38 (1.02–5.54), p=0.04 | 2.38 (1.02–5.55), p=0.04 | 2.37 (1.02–5.54), p=0.04 |
Abbreviations: SOFA, Sequential Organ Failure Assessment; PaO2, arterial oxygen partial pressure; FiO2, fraction of inspired oxygen.
This study showed that following a structured protocol to reduce FiO2 based on SpO2 was associated with reduction of hyperoxemia on day 1 and sustained hyperoxemia. Moreover, excess oxygen use was lower in the conservative-oxygen ICU compared with control ICU, and this reduction was not associate with hypoxemia occurrence. We found no relationship between hyperoxemia or excess oxygen use and hospital mortality in our cohort of COVID-19 under mechanical ventilation.
Other studies have demonstrated that hyperoxemia occurs in mechanically ventilated patients and that higher FiO2 than necessary is set in these patients. A retrospective study that evaluated patients under MV showed that 49.8% presented hyperoxemia during the first 24h of MV, among whom the mean FiO2 was 62%.13 The LUNG SAFE study, a prospective cohort of patients with ARDS under MV, showed that 30% of them presented hyperoxemia on day 1 of MV, and 12% sustained hyperoxemia on days 1 and 2 of MV. Among patients with hyperoxemia, 66% were ventilated with FiO2 higher than 60%.7
The multivariable analysis also showed that being admitted in the conservative-oxygen ICU was associated with lower occurrence of hyperoxemia. This result suggests that following a structured protocol of FiO2 adjustment reduces oxygen use and the occurrence of hyperoxemia, without increasing the risk of hypoxemia, which was not observed in any of the patients in the two ICUs on days 1 and 2. A possible reason for the excessive use of oxygen and the high number of hyperoxemic patients during MV might be that doctors are more worried about hypoxemia than hyperoxemia. Therefore, FiO2 reduction tends to be avoided. Other factors might have contributed to the excess use of oxygen, especially during the COVID-19 pandemic. The massive number of patients mechanically ventilated in the ICUs during the pandemic resulted in work overload for the health care professionals. This fact may have reduced the frequency in which the ventilatory parameters were adjusted. Moreover, less specialized staff worked in the ICUs during the pandemic, with limited training in MV and limited knowledge of the risk of hyperoxemia.
In the present study, neither hyperoxemia, nor excess oxygen use were independently associated with mortality. Our results with ARDS COVID-19 patients are in accordance with those found in the LUNG SAFE study, in which ARDS non-COVID-19 patients were assessed.7 Recently, two randomized clinical trials (LOCO2 and HOT-ICU) also failed to show differences in mortality in hypoxemic acute respiratory failure patients who underwent conservative or liberal oxygen therapy.9,10 A post hoc analysis of the HOT-ICU trial with COVID-19 patients only did not show a statistically significant difference in mortality between a lower and a higher oxygenation target.14 Conversely, an analysis of the results of 10 trials conducted by the ARDS Network showed that higher oxygen exposure, defined as FiO2 higher than 0.5 with PaO2 higher than 80mmHg was associated with lower ventilator-free days and higher mortality.15 The reasons for those conflicting results might include different clinical characteristics and severity among included patients, who may have different oxygen demands, and different levels of hyperoxemia that occurred in the studies. Negative impact on patients outcomes might occur as a result of higher levels or more extended periods of exposure to hyperoxemia.16,17 Those results showed that a more conservative oxygen use in mechanically ventilated patients is feasible, safe, and can reduce the harmfulness associated with hyperoxemia. Moreover, this strategy reduces costs and spare oxygen, a gas that became scarce in some regions during the COVID-19 pandemic.
This study has several limitations. 1. Patients were not randomized to the groups but selected according to the ICU where they were admitted. Therefore, confounding variables other than admission in an ICU with a FiO2 protocol might have contributed to the occurrence of hyperoxemia and excessive oxygen use. 2. Since a convenience sample was used, without sample size calculation, this study may have had limited power to detect associations between hyperoxemia or excess oxygen use and mortality. 3. Data were collected once, at a standardized time each morning, on the first two days of MV. Consequently, these data may not properly reflect neither the different values of FiO2 and PaO2 over the course of those days, nor those values over the following days of MV. 4. Data were obtained in only two ICUs, which limits the extrapolation of the results to other ICUs.
In conclusion, our findings showed that hyperoxemia and excess oxygen use may be prevalent in COVID-19 patients mechanically ventilated and that following FiO2 adjustment protocol can reduce those two events. Even though hyperoxemia and excess oxygen use were not associated with worse clinical outcomes, adopting a FiO2 protocol is safe and may be useful in a scenario of depleted oxygen resources, as was seen during the COVID-19 pandemic.
Author contributions- -
Edimar Pedrosa Gomes: conception and design of the study; acquisition of data; analysis and interpretation of data; drafting the article; final approval of the version to be published.
- -
Maycon Moura Reboredo: conception and design of the study; analysis and interpretation of data; drafting the article; final approval of the version to be published.
- -
Giovani Bernardo Costa: acquisition of data; analysis and interpretation of data; revising the article, providing intellectual content of critical importance to the work described; final approval of the version to be published.
- -
Fabrício Sciammarella Barros: acquisition of data; analysis and interpretation of data; revising the article, providing intellectual content of critical importance to the work described; final approval of the version to be published.
- -
Erich Vidal Carvalho: conception and design of the study; acquisition of data; drafting the article; final approval of the version to be published.
- -
Bruno Valle Pinheiro: conception and design of the study; analysis and interpretation of data; drafting the article; final approval of the version to be published.
This research did not receive any specific grant from funding agencies in the public, commercial, or not-for-profit sectors.
Conflict of interestNone.