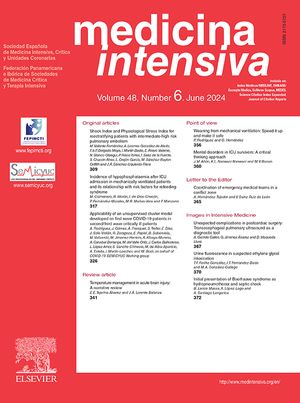
Edited by: Ana Ochagavía - Hospital Universitario de Bellvitge. L'Hospitalet de Llobregat. Barcelona. Spain.
More infoTranscranial ultrasonography is a non-invasive, bedside technique that has become a widely implemented tool in the evaluation and management of neurocritically ill patients. It constitutes a technique in continuous growth whose fundamentals (and limitations) must be known by the intensivist. This review provides a practical approach for the intensivist, including the different sonographic windows and planes of insonation and its role in different conditions of the neurocritical patients and in critical care patients of other etiologies.
La ultrasonografía transcraneal es una técnica no invasiva y disponible a pie de cama que se ha convertido en una herramienta accesible y consolidada en la evaluación y el manejo clínico de los pacientes neurocríticos. Se trata de una técnica en continuo crecimiento cuyos fundamentos (y sus limitaciones) deben ser conocidos por el intensivista. Esta revisión aporta un enfoque práctico para el intensivista, incluyendo las diferentes ventanas y planos de insonación y su papel en la patología específica propia de los pacientes neurocríticos y en los pacientes críticos de otras etiologías.
The use of ultrasound has gained popularity in the Intensive Care Units (ICUs) of our setting, and the technique currently forms a routine part of the evaluation and management of critical patients. In this respect, the European Society of Intensive Care Medicine (ESICM) has published a consensus document on the competencies required of intensivists in relation to critical care in general.1 More specifically, the competencies referred to the use of transcranial ultrasound in the ICU have also been published.2
The first descriptions of the use of transcranial Doppler ultrasound (TCD) for the assessment of cerebral artery flow velocities in neurocritical patients were documented in 1982 by Aaslid et al.3 With the incorporation of technological improvements, ultrasound systems also allowed analysis of the cerebral parenchyma in addition to color visualization of the basal venous and arterial vessels, and doppler spectral analysis – all in real-time, at the patient bedside, and in a non-invasive manner using transcranial color-coded duplex (TCCD) protocols. The expansion of technical capabilities, improved knowledge of the technique, and its increased incorporation into ICUs have made it possible to broaden these applications to different types of critical patients.5 In general, TCCD is performed using a 2–2.5MHz probe that allows visualization of the main brain structures, as well as the analysis of cerebral hemodynamics.5
The basic ultrasound exploration windows and their main applications are summarized below:
- -
Temporal: Identification of the mesencephalon and ventricular system, evaluation of midline deviation (MLD), and exploration of the main arteries of the circle of Willis.
- -
Suboccipital or transforaminal: Identification of the foramen magnum, with analysis of the vertebral arteries and the basilar artery.
- -
Transorbital: Exploration of the ophthalmic artery and carotid siphon, as well as evaluation of the diameter of the optic nerve sheath (ONSD).
- -
Submaxillary: Exploration of the extracranial portion of the internal carotid artery, of use in calculating the Lindegaard ratio.
In general, any exploration should begin with an analysis of the temporal window. This window has three fundamental exploratory planes:
Mesencephalic plane: We recommend exploration at an initial depth of 15−16cm, in order to check the correct insonation of the contralateral dome (usually at about 14cm from the transducer in adults). At the center of the image thus obtained we can identify the mesencephalon with its characteristic butterfly wing-shaped hypoechoic appearance.
Diencephalic plane: Angulation of the transducer 10° in the cranial direction allows us to identify the third ventricle as a pulsatile hyperechoic double parallel line located anterior to the mesencephalon.
Ventricular plane: Further angulation of the transducer 10° in the cranial direction allows visualization of the thalamus and frontal horns of the lateral ventricles.
In all cases, the existence of a bone defect or decompressive craniotomy increases the performance of the exploration. From these planes, we can further examine the clinical applications of the two-dimensional (2D) study:
Midline deviation: One of the factors systematically associated with a poorer neurological outcome in neurocritical patients is the presence of a mass effect, evaluated using MLD. Many studies have demonstrated an excellent correlation between the assessment of MLD with computed tomography (CT) and with TCCD performed at the patient’s bedside.6–8 The evaluation of MLD is made from the temporal window, guiding the ultrasound beam perpendicular to the walls of the third ventricle (3V), which appears as a double hyperechoic line at the center of the plane, and measuring the distance from the transducer to the center of the 3V bilaterally (distances A and B). We then apply the formula: MLD=A-B/2 (Fig. 1). In patients with decompressive craniotomy, Caricato et al. described a specific method that affords good results.9 In these cases, the interventricular line, defined as the line between the lateral ventricles, is traced with the ultrasound device. We then trace a new line from the frontal point to the occipital point of the falx cerebri. The distance between the two lines represents the MLD.9
Dilatation of the ventricular system: The third ventricle appears in the diencephalic plane as a hyperechoic double parallel line (Fig. 2a). The frontal horns of the lateral ventricles are likewise visualized in this way (Fig. 2b). In both cases, the content is anechoic, unless intraventricular hemorrhage has occurred.5 Different studies have found an excellent correlation between CT and TCCD in measuring the diameter of the third ventricle10,11 and the frontal horns,12 making it even possible to assess the response to cerebrospinal fluid (CSF) drainage.12 Kiphuht et al. estimated that a cut-off point of under 5.5mm in the increase in ventricular diameter after clamping of the drain exhibits a sensitivity of 100% and a specificity of 83%, with a negative predictive value of 100%, in reference to the safe withdrawal of drainage.12 This is probably the main consideration of the application, and its evolutive behavior should be evaluated. In the case of measurements of the anterior horns, the angle of insonation must be taken into account to minimize the risk of erroneous measurements.13 In experienced hands, this procedure may be useful even for assessing the normal or abnormal positioning of the ventricular drain and for alerting us to the need for additional tests.5
Intraparenchymal/extraaxial hematomas: In the acute phase (<10 days), intraparenchymal blood appears hyperechoic. This makes it possible to distinguish it from ischemic lesions which are hypoechoic and moreover present other indirect signs such as the identification of arterial occlusion, the presence of collateral flows, diminished pulsatility of the infarction zone, and a mass effect as measured by MLD.4,5,14 In a series of 151 patients of which 12% were excluded due to a poor acoustic window, Mäurer et al. reported a concordant diagnosis between transcranial ultrasound and CT in 95% of the cases.15 In addition, no complications that may have required additional treatments were identified.15 In a series of 34 patients with large hematomas (86±45ml) in the first three hours, Santamarina-Pérez et al. observed an excellent correlation in monitoring the increase in hematoma in terms of all diameters and total volume, with a correlation coefficient of 0.888 (95%CI 0.8−0.937).16 Thus, an early study with daily monitoring is recommended to assess the increase in hematoma and thus avoid daily patient transfer to the CT room.14
The use of TCCD also allows the diagnosis of extra-axial, epidural or subdural hematomas,17,18 though apart from the morphological characteristics, it is difficult to distinguish among them.4
Evaluation of cerebral hemodynamicsBasic conceptsTranscranial ultrasound allows us to evaluate cerebral hemodynamics from the determination of blood flow velocity parameters of the large intracranial vessels.14 This is done based on the Doppler effect, which can monitor red cell movement within the explored blood vessel. The equation derived from this principle constitutes the basis for calculating cerebral blood flow (CBF) velocity, though it assumes a constant vessel diameter. The insonation angle is taken to be constant in transcranial Doppler ultrasound (TCD)3 and can be adjusted to the trajectory of the explored vessel in TCCD; this gives rise to some adjustments and increased precision in determining the velocities,19 particularly in those cases with an insonation angle of over 30°.14
Adequate insonation of the intracranial vessel can yield a pulse wave with which to determine flow velocities (systolic (Vs), diastolic (Vd) and mean (Vm)) and calculate the pulsatility index (PI), resistance index (RI) and Lindegaard ratio (LR).
The normal velocity ranges of each artery were described many years ago,3 though many factors influence these values, such as patient age and gender, partial pressure of carbon dioxide, blood pressure (BP) or intracranial pressure (ICP).5 Systolic flow velocity is mainly dependent upon cardiac output. In contrast, Vd could be regarded as a cerebral hypoperfusion index, since an increase in ICP produces a decrease in cerebral perfusion pressure (CPP), reflected in a decrease in diastolic flow. Lastly, Vm is correlated to CBF and is mainly used to estimate cerebral arterial flow.3
On the other hand, the PI of Gosling has possibly been the most widely used cerebral hemodynamic parameter. It is obtained from the formula (Vs – Vd)/Vm.5 The main advantage of this parameter is that it is not directly affected by the angle of insonation. The normal values range from 0.5 to 1–1.4, and are affected by many parameters such as heart rate, BP, ICP or temperature.5,20 The PI informs us about changes in cerebral hemodynamics, but should not be used isolatedly for assessing cerebral vascular resistance or ICP.20,21
Cerebral vasospasmCerebral vasospasm is one of the key elements in the appearance of late neurological deterioration in patients with aneurysmal subarachnoid hemorrhage. The use of transcranial ultrasound for detecting arterial narrowing reflected by an increase in the recorded velocities constitutes a common practice in most ICUs in our setting, particularly in application to the middle cerebral artery (MCA). According to a recent systematic review, the sensitivity, specificity and positive and negative predictive values of TCD are 66.7%, 89.5%, 93.7% and 53.4%, respectively, and these figures increase in the case of TCCD to 81.5%, 96.6%, 98.2% and 69.1%.22
Traditionally, the cut-off points used in the MCA have been 120cm/s and 200cm/s, with the former value being more sensitive and the latter more specific. These recordings are to be complemented by the measurement of LR, which represents the ratio between the velocity in the MCA and the ipsilateral internal carotid artery in its extracranial portion.23 This ratio allows us to differentiate situations of hyperafflux (LR<3) and severe vasospasm (LR>6).24 Accordingly, Vm in the MCA<120cm/s has a negative predictive value of 94%, while Vm>200cm/s has a positive predictive value of 87%. In the case of the basilar artery (BA), a ratio of the Vm between the BA/extracranial vertebral artery>2 has a sensitivity of 73% and a specificity of 80% in diagnosing vasospasm.24 However, it must be noted that while these values are static, both aneurysmal subarachnoid hemorrhage and vasospasm are dynamic phenomena. Consequently, some authors suggest that significant Vm elevation in 24h is the best ultrasound indicator of significant arterial narrowing even in the first days after aneurysmal subarachnoid hemorrhage.25 This may also prove useful in assessing treatment response (Fig. 3).
More recently, and with the introduction of the study of the main cerebral veins and their correlations to global cerebral blood flow, a new parameter has been described: the ratio between Vm in the MCA and the basal vein of Rosenthal (intracranial arteriovenous index (AVI)). In this respect, AVI>10 for Vm and AVI>12 for Vs have been shown to offer a precision of 87% and 86%, respectively, which is slightly better than with the use of LR.26
Cerebral autoregulationThe assessment of cerebral autoregulation can be made at the patient’s bedside with transcranial ultrasound, allowing us to analyze both the static and dynamic components of autoregulation. The static rate of regulation (sROR) evaluates the response of CBF to changes in CPP, and is measured as the ratio between the percentage change in cerebrovascular resistance (CVR) and the percentage change in CPP, where CVR=mean blood pressure/flow velocity.14
On the other hand, the evaluation of dynamic autoregulation can be made using techniques such as the transient hyperemia response test. The latter is based on the compensatory arteriolar vasodilatation that occurs after brief external compression of the common carotid artery.14,27 In patients with preserved autoregulation, Vs is seen to increase by over 9% after carotid compression. Both carotid axes should be tested.14,24 Evaluation can also be made by analyzing the spontaneous changes in blood pressure and CPP over time while measuring CBF velocity. In this regard, several parameters have been described, such as Mx (mobile correlation coefficient between Vm and CPP), Mxa (correlation coefficient between Vm and blood pressure) and the autoregulation index (ARI), an adimensional index that ranges between 0 and 9, for which estimation is made of the response of CBF velocity to a hypothetical change in blood pressure based on analysis of the transfer function of the spontaneous fluctuations in blood pressure and CBF velocity.28–30
Detection of shuntsTranscranial ultrasound allows early evaluation of the presence of a patent foramen ovale or of pulmonary arteriovenous shunts in the etiological diagnosis of ischemic stroke.24 For the detection of microbubbles it is necessary to inject a gaseous contrast or prepare a 10-ml syringe containing 9ml of saline solution and 1ml of air shaken together to generate microbubbles. These microbubbles displace from the right to the left circulation in the cardiac cycle, enter the systemic circulation, and can be detected by TCD due to the fact that their greater acoustic impedance generates signals known as HITS (high-intensity transient signals) or MES (microembolic signals) of high intensity (>3dB) and short duration (<300ms), appearing above the ultrasound baseline with a characteristic “click” sound, and which are independent of the cardiac cycle.
The study should be repeated by inducing Valsalva maneuvers to increase the precision, which in experienced hands is very close to that of transesophageal echocardiography (the test of choice). Transcranial ultrasound is therefore particularly interesting in the context of screening.31
Screening of intracranial hypertensionThe use of transcranial ultrasound in the screening of intracranial hypertension (ICHT) is particularly interesting in situations characterized by limited resources, and can be made in different ways, as described below.
Measurement of the diameter of the optic nerve sheath (ONSD)The optic nerve is covered by the meningeal layers, in the form of an extension of the cerebral subarachnoid and subdural space. Thus, when ICP increases, the cerebrospinal fluid displaces towards this space, increasing the diameter of the optic nerve, particularly in the bulbous portion, which is more distensible and is located 3mm from the retina and optic disc.32,33
A recent meta-analysis including 18 prospective studies evidenced a sensitivity of 90% and a specificity of 85% in detecting ICHT, with a correlation coefficient between invasive ICP monitoring and ONSD of 0.7 (95%CI 0.63−0.76; P<.05).32 There is no exact cut-off point indicative of ICHT, though most studies suggest cut-off points of 5−6mm.5 The combined measurement of ONSD with Vs of the sinus rectus increases the diagnostic precision of the technique.34 The association of ONSD with radiological parameters related to ICHT is also of interest, especially in contexts where invasive ICP monitoring is not available.35–37
Although ONSD appears to increase within a few minutes after an increase in ICP, it is not clear whether the measurement of ONSD is able to reflect the dynamic changes in a precise manner.38,39 Ocular trauma is the only absolute contraindication to ultrasound ONSD assessment. On the other hand, it must be taken into account that the artifact produced by the central artery of the retina can give rise to interpretation error, since this artery runs close and in parallel to the nerve, and can generate a hypoechoic image that is difficult to distinguish from the optic nerve.24,40
Intracranial pressure according to cerebral hemodynamicsThe pulsatility index (PI) has been the single most widely used hemodynamic parameter for evaluating the existence of ICHT, since the circulatory flow resistances increase with rising ICP.3,4 In this regard, Tamagnone et al. have proposed a severe traumatic brain injury (TBI) resuscitation protocol guided by a PI cut-off point of >1.441; however, although PI affords information, we feel that it should not be used isolatedly in the evaluation of ICP except in extreme values20,21 but rather within a multimodal monitoring context (Fig. 4). A recent meta-analysis evaluating methods for the prediction of ICHT showed PI to have poor performance in determining the increase in ICP (area under the ROC curve 0.55−0.72),42 mainly due to the fact that PI is dependent upon multiple physiological variables.5,20
Nevertheless, different studies including patients with mild or moderate TBI have established a close association between the increase in PI and the decrease in diastolic velocities, with the possibility of neurological deterioration and poor final outcomes – concluding that TCD is a useful complementary tool for triage and follow-up of individuals of this kind.43–45
More recently, the multicenter IMPRESSIT-2 trial analyzed the estimation of ICP through insonation of the middle cerebral artery in 262 neurocritical patients using the following formula based on the results obtained in a previous pilot study and comparing it against invasive measurement46,47:
where CPPtcd=MABP×Vd/Vm+14The negative predictive value of this way of estimating ICP was high for all the ICP cut-off points evaluated invasively (ICP>20mmHg=91.3%; ICP>22mmHg=95.6% and ICP>25mmHg=98.6%). The optimum cut-off point of this index was 20.5mmHg, exhibiting a sensitivity of 70% (95%CI 40.7%–92.6%) and a specificity of 72% (95%CI 51.9%–94.0%), with an area under the ROC curve of 76% (95%CI 65.6%–85.5%).44 The concordance between ICPtcd and invasive ICP was 33.3% (95%CI 25.6%–40.5%), with a shift of −3.3mmHg. Considering all these values, the authors concluded that transcranial ultrasound has a high negative predictive value in the screening of ICHT and thus may be particularly interesting in settings characterized by limited resources or in patients in which invasive monitoring is contraindicated, or as a screening method in cases of uncertainty.46
Cerebral circulatory arrestTranscranial ultrasound is a useful tool for detecting cerebral circulatory arrest (CCA) accompanying brain death – two evolutive processes that do not always coincide in time.48,49 As a general norm, CCA lies at the extreme and devastating end of ICHT, especially in patients with supratentorial injuries. Thus, the ultrasound patterns follow the evolutive changes of the increase in ICP, characterized by high resistance patterns in crescendo until the definitive cessation of cerebral blood flow.50,51
Before ultrasound exploration is made, we must ensure the necessary requirements including hemodynamic stability, normal oxygenation, normocapnia and normothermia.48 Furthermore, it is advisable to measure the MCA bilaterally and the basilar artery in two explorations spaced 30min apart in order to avoid false positive readings.52
Four patterns are classically recognized49,50 (Fig. 5):
- •
Absence of diastolic flow: Diastolic flow is basically dependent upon the vascular resistances, which in turn are mainly determined by ICP and the diameter of the vessel. This pattern is seen when ICP equals the diastolic blood pressure, where flow at the end of the diastole is zero. It reflects severe ICHT but is not correlated to a situation of CCA in arteriography.
- •
Reverberant or oscillating flow: This manifests when the ICP levels are equal to or greater than systolic blood pressure. At this moment cerebral perfusion ceases. It is represented as waves that exhibit anterograde systolic flow and retrograde or inverted diastolic flow. This pattern is correlated to CCA in arteriography.
- •
Systolic spicules, peaks or spikes: ICP clearly exceeds systolic blood pressure. Short systolic waves (<200ms) of low velocity (systolic peak<50cm/s) are produced. No diastolic flow is noted. Low-level filters (50Hz) are needed for detection. This pattern is correlated to CCA in arteriography.
- •
Absence of signal: This is seen in very advanced phases in time and with very high ICP. In this context, it may be considered that the absence of signal may be due to the absence of an acoustic window. Consequently, in order to accept this pattern as being diagnostic of CCA, the exploration must have been made by the same operator and under the same conditions as a previous exploration demonstrating sufficient acoustic window and preserved flow. In order to improve the performance of the exploration, we can also use echo-enhancers, thereby increasing the number of insonated vessels and conclusive studies.53
A recent meta-analysis has evidenced that transcranial ultrasound has a sensitivity of 90% and a specificity of 98% in diagnosing CCA.54 False positive readings may occur in patients with a shock that do not meet the prerequisites or in situations of a sudden rise in ICP, such as the existence of A waves or subarachnoid bleeding at the time of aneurysmal rupture. All this underscores the need for a rigorous exploration performed by expert operators under adequate conditions. On the other hand, there may be persistent flow despite an exploration suggestive of brain death (false negatives) in cases of a lack of cranial sealing (decompressive craniotomy, dome fractures, cranial base fractures, open fontanelles, ventriculotomy) and in patients with other brain damage mechanisms such as infratentorial lesions and anoxic-ischemic encephalopathy.54,55
Use in non-neurological critical patientsIn recent decades, new applications have been described in non-neurological critical patients, including the monitoring of CBF during cardiopulmonary resuscitation (CPR) following cardiac arrest and after recovery of spontaneous circulation (RSC), as well as applications in patients with critical liver disease.
Cardiac arrest and following recovery of spontaneous circulationTranscranial ultrasound can prove useful in cardiopulmonary resuscitation and after recovery of spontaneous circulation. During CPR, the technique can be used to monitor the effect of chest compressions upon cerebral perfusion. In this context, the ideal vessel for exploration is the siphon of the internal carotid artery through the transorbital window,56 though in no case should exploration be allowed to complicate CPR maneuvering.5 This strategy allows us to observe the resolution of cardiac arrest by evidencing an increase in CBF velocity, as well as the appearance of the diastolic component of the spectrum, even before identifying the presence of a peripheral arterial pulse.57
Following RSC, transcranial ultrasound is not a precise tool for assessing the neurological outcome,5 though it can be useful in hemodynamic and ventilatory optimization and for ensuring adequate cerebral perfusion.58 In general terms, following RSC, three patterns that tend to normalize in the first 72h can be seen57:
- -
Normal hemodynamic pattern, which is generally associated with a good prognosis.
- -
Hypodynamic pattern (low Vm and high PI), reflecting hypoperfusion secondary to thrombosis and vasospasm of the cerebral microcirculation, hypoperfusion secondary to cell and capillary edematization, or both.
- -
Hyperdynamic pattern (elevated Vm and low PI with LR<3), which beyond 30min is associated with a poorer prognosis and the development of intracranial hypertension.
Furthermore, as in other populations, the loss of cerebral autoregulation is consistently correlated to a poorer neurological outcome.59
Patients with liver failureThe fundamental role of transcranial ultrasound in this patient population is the screening of ICHT in the context of fulminant liver failure. The routine invasive monitoring of ICP has not shown benefits in terms of patient survival, and on the other hand, may result in fatal bleeding complications. Thus, the use of transcranial ultrasound, estimating ICP and measuring ONSD, may allow us to identify those patients presenting ICHT and who require invasive monitoring. However, in this population, the technique appears to be less precise than in other neurocritical patient populations.5
On the other hand, it is of interest to mention that hyperemia (which tends to precede ICHT) is common in this patient population, and that autoregulation disorders are associated with a poorer prognosis5 - though recovery can be rapid once liver function is restored.60
Another application in this context is the identification of intrapulmonary vascular dilatation (IPVD) in patients with liver cirrhosis. In this sense, the findings of transcranial ultrasound with contrast are similar in terms of the detection of late right-left shunting with recirculation to those obtained using echocardiography with contrast, which is regarded as the technique of choice.61
ConclusionsIn summary, transcranial ultrasound is a noninvasive technique that can be used at the patient’s bedside and which offers a broad range of applications in the monitoring of critical patients. The technique is under constant evolution, and intensivists should be familiarized with its principles (and limitations).
Author contributionsLlompart-Pou, Galarza, Amaya-Villar and Godoy participated in writing of the first version of the manuscript. Llompart-Pou and Godoy produced the figures of the manuscript. Llompart-Pou drafted the submitted final version of the manuscript, which was approved by all the authors.
Conflicts of interestThe authors declare that they have no conflicts of interest.
FundingNone.