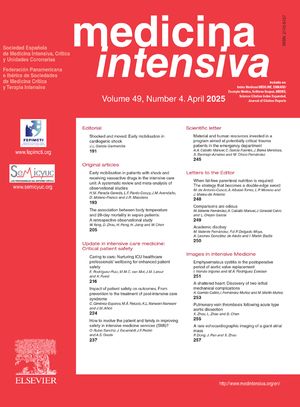
Edited by: Federico Gordo - Medicina Intensiva del Hospital Universitario del Henares (Coslada-Madrid)
Last update: February 2024
More infoThe rapid increase in antibiotic(ATB) resistance among Gram-negative bacilli(BGN), especially in strains of Enterobacteriaceae, Pseudomonas aeruginosa, and Acinetobacter baumannii, with high resistance patterns (XDR), poses a huge threat to health systems worldwide.
In the last decade, different ATBs have been developed against XDR, some of which combine a lactam β along with a β-lactamase inhibitor, while others use non-β-lactam inhibitors. Most of them have adequate “in vitro” activity on several β-lactamases of class A, C and D of Ambler. However, combinations such as Ceftazidime/avibactam, Ceftolozane/Tazobactam and Meropenem/vaborbactam have no activity against metallo-β-lactamases(MβL). New combinations such as Aztreonan/AVI, Cefepime/Zidebactam, or new cephalosporins such as Cefiderocol, have efficacy against MβL enzymes. Although some of these combinations are already approved and in the commercialization phase, many of them have yet to define their place within the treatment of microorganisms with high resistance through clinical studies.
El rápido incremento en las resistentes a los antibióticos (ATB) entre los bacilos Gram negativos (BGN), especialmente en cepas de Enterobacterias, P. aeruginosa, y A.baumannii, con elevadas elevados patrones de resistencia (XDR), plantea una enorme amenaza para los sistemas de salud en todo el mundo.
En la última década, diferentes ATB han sido desarrollados contra XDR, algunos de los cuales combinan un β-lactámico junto con un inhibidor de β-lactamasa, mientras que otros utilizan inhibidores no β-lactámicos. La mayoría de ellos presenta una adecuada actividad “in vitro” sobre varias β-lactamasas de clase A, C y D de Ambler. Sin embargo, combinaciones como Ceftazidime/avibactam, Ceftolozano/Tazobactam y Meropenem/vaborbactam no presentan actividad contra metalo-β-lactamasas (MβL). Nuevas combinaciones como Aztreonan/AVI, Cefepime/Zidebactam, o modernas cefalosporinas como Cefiderocol, presentan eficacia contra casi la totalidad de las MβL. Aunque algunas de estas combinaciones ya están aprobadas y en fase de comercialización, muchas de ellas aún deben definir su lugar dentro del tratamiento de microorganismos con resistencia elevada a través de estudios clínicos.
Antimicrobial resistance is a reality and a constant concern for healthcare professionals, given the lack of availability of new molecules to treat highly resistant microorganisms.1–3 This problem has been exacerbated by the virulent pandemic caused by severe acute respiratory syndrome due to coronavirus type 2, resulting in COVID-19, which has, at the time of writing this review, caused more than 350 million infections, with just over 5 million deaths worldwide. This situation has not only created a crisis in the global healthcare system, but has also led to an uncontrolled increase in superinfections in patients with COVID-19, with a high and possible excessive use of antibiotics (ATB). According to data from the ENVIN-HELICS registry (https://hws.vhebron.net/envin-helics/), the incidence density of ventilator-associated pneumonia (VAP) rose from just over 5 episodes/1000 mechanical ventilation (MV) days in 2019 to 11.5 episodes/1000MV days during the pandemic (2020), while ATB use in intensive care unit (ICU) patients grew from 61% (2019) to more than 90% during the pandemic (2020). At the same time, the isolation of multidrug-resistant gram-negative bacilli (GNB) was up by just over 40% overall, but if carbapenemase-positive GNB are considered, the observed incidence doubled with respect to 2019 from 0.8% to 1.66% overall and, especially, the acquisition of these microorganisms in the ICU tripled, from 0.35% to 1.33% in 2020.
Although the definition of bacterial resistance has been modified in recent years, we can assume that three types of microbial resistance are defined: 1) multidrug-resistant (MDR), defined as resistance to at least one agent in three or more ATB families; 2) extensive drugs resistance (XDR), defined as resistance to at least one ATB in all but one or two of the families, and 3) pan-drug resistance (PDR), defined as resistance to all ATBs. Recently, a new category called difficult-to-treat resistance (DTR) has been recognised, defined as high resistance to first-line ATBs.4,5 Semantic issues aside, infections caused by resistant microorganisms are associated with diminished quality of life, increased health costs, the need for more expensive ATBs or the reuse of «old» ATBs having a high-toxicity profile, and, of course, increased mortality.6,7
To complicate the picture even further, development programmes for new ATB agents are conditioned by a strong disincentive for companies, as the return on investment in ATB development is significantly lower than for other drugs for chronic diseases or cancer.8,9 If one considers the observed mortality and treatment-related economic impact, the group of micro-organisms called «ES[KC]APE» (E: Enterococcus faecium; S: Staphylococcus aureus or also Stenotrophomonas maltophilia; K: Klebsiella pneumoniae, or, recently, C: Clostridioides difficile; A: Acinetobacter baumannii; P: Pseudomonas aeruginosa; E: Enterobacter spp or recently Enterobacteriaceae) pose the most formidable clinical challenge today.10,11 To counter this adverse scenario, the European Federation of Pharmaceutical Industries and Associations partnered with the European Union to establish the largest public-private partnership for the development of the Innovative Medicines Initiative under the New Drugs for Bad Bugs programme, which encourages everything from new antibiotic development and clinical research to the reshaping of antibiotic use, in the hope of catalysing the approval of new antibiotics.12
The aim of this review is to gain insight into clinically relevant aspects of new ATBs and their combinations for the treatment of resistant GNBs. To this end, a Pubmed/Medline search was conducted from 2012 to 2021 using the keywords: intensive care, critical care, nosocomial pneumonia, hospital-acquired pneumonia, healthcare-associated/-acquired pneumonia, ventilator-acquired pneumonia, in combination with novel antibiotics/antimicrobials, ceftazidime/avibactam, ceftolozane/tazobactam, imipenem/relebactam, meropenem/vaborbactam, cefepime/zidebactam, aztreonem/avibactam, cefiderocol. The review includes the results of randomised studies, meta-analyses, high-quality observational studies, systematic or narrative reviews, international guidelines, and expert opinions. Due to the limited scope of this review, not all ATBs in the pipeline can be included; consequently, those marketed or close to approval and with special indication in respiratory infection have been chosen.
B-Lactams+β-lactamase inhibitorCeftazidime/avibactam (CAZ/AVI)CAZ is a third-generation cephalosporin with activity against Pseudomonas spp. combined with a semisynthetic non-β-lactam β-lactamase inhibitor (AVI), which has already been marketed and approved for the treatment of severe GNB infections.13 The combination with AVI restores the effectiveness of CAZ on β-lactamase-producing bacteria (BLEE, AmpC, and CPE), such as Enterobacteriaceae and Pseudomonas aeruginosa (Fig. 1). AVI, together with CAZ, reduces the minimum inhibitory concentration (MIC50 and MIC90) against P. aeruginosa, possibly due to inactivation of AmpC. However, the additional resistance mechanisms that Pseudomonas spp. present, such as alterations of porins, metallo-β-lactamases (MBL), efflux pumps, and OXA β-lactamases, among others, mean that the effectiveness of this ATB is more evident in enterobacteria. CAZ/AVI demonstrates high efficacy against MDR and XDR enterobacteria compared to its comparators, such as meropenem (MERO) or piperacillin/tazobactam (PTZ).14 In a recent systematic review by Soriano et al.15 involving 1926 patients treated with CAZ/AVI (alone or in combination) and 1114 patients as comparators/controls, the authors observed a favourable outcome associated with CAZ/AVI in patients with severe GNB infections. The most common infections reported among the infected participants were pneumonia, bacteraemia, and skin and soft tissue infection (SSTI). It is interesting to note that 1718 Enterobacteriaceae exhibited carbapenem resistance or carbapenemase production and 150 P. aeruginosa had MDR and XDR among the patients treated. Most of the publications included in the review reported a more favourable outcome for CAZ/AVI (clinical cure between 45% to 100%) and statistically superior to comparator ATBs. On the other hand, the authors point out that resistance was uncommon, and generally in carbapenemase-producing Klebsiella pneumoniae (KPC). The authors conclude that the review provides evidence of sufficient quality to support the use of CAZ/AVI in the treatment of hospitalised patients with carbapenemase-producing Enterobacteriaceae and MDR P. aeruginosa.
Action of new antimicrobials on different beta-lactamases alone or in combination.
X not active, √ active. Variable activity: 1 for P. aeruginosa, 2 for Enterobacteriaceae.
ATZ/AVI: aztreonam/avibactam; CAZ/AVI: caftazidime/avibactam; CFD: cefiderocol; CFP/TBB: cefepime/taniborbactam; CFP/ETZ: cefepime/enmetazobactam; CFP/ZDB: cefepime/zidebactam; CTZ/TAZ: ceftolozane/tazobactam; IMI/CIL/REL: Imipenem/cliastatin/relebactam; MERO/NACU: meropenem/nacubactam; MERO/VABO: meropenem/vaborbactam.
In summary, CAZ/AVI inhibits Ambler class A and C β-lactamases and some class D enzymes, including broad-spectrum β-lactamases (BLEE), KPC and OXA-48 carbapenemases, and AmpC enzymes. CAZ/AVI does not inhibit class B enzymes (metallo-β-lactamases) and fails to inhibit many class D enzymes. Table 1 provides comparative characteristics between CAZ/AVI and other combinations.
Distinguishing characteristics of the main combinations on the market.
Characteristics | CAZ/AVI | CTZ/TAZ | MERO/VABO | IMI/CIL/REL | CFD |
---|---|---|---|---|---|
Half-life (h) | 1.5−3.0 | 1.0−2.6 | 1.0−1.9 | 1.1−1.8 | 2−2.7 |
Protein binding (%) | 5−22 | 16−30 | 2−33 | 20−22 | 58 |
Distribution volume (l) | 19−28 | 12−19 | 19−29 | 19−22 | 16 |
Lung penetration (AUC ELF/AUC plasma) | 0.31/0.35 | 0.44−0.48 | 0.58−0.3 | 0.43−0.44 | 0.1 |
Elimination | Renal | Renal | Renal | Renal | Renal |
Indications | |||||
GNB with limited treatment options | Yes | No | Yes | Yes | Yes |
Complicated IIA | Yes | Yes | Yes | Yes | No |
NP | Yes | Yes | Yes | Yes | Yes |
Secondary bacteraemia | Yes | No | Yes | Yes | No |
Administration | 2g/0.5g every 8h | 2g/1g every 8h | 2g/2g every 8h | 0.5g/0.5g/0.25 every 6h | 2g every 8h |
Duration of infusion (h) | 2 | 1 | 3 | 1 | 3 |
Adjustment for hyperfiltering renal function | No | No | No | No | Yes (>120) 2g every 6h |
Adjustment for AKI (CrCL cut-off in mL/min) | Yes (<50) | Yes (<50) | Yes (>40) | Yes (<90) | Yes (<90) |
30−50=1g/0.25g every 8hb | 30−50=1g/0.5g every 8ha | 39−20=1g/1g every 8hc | 90−60=400/400/200 every 6hd | 90−60=2g every 8he | |
15−29=0.75g/0.1875g every 12hb | 15−29=0.5g/0.25g every 8ha | 10−19=1g/1g every 12hc | 59−30=300/300/150 every 6hd | 59−30=1.5g every 8he | |
HD=0.75g/0.1875g every 24−48hb | HD=1.5g/0.75g loadinga+300mg/150mg every 8h post-HD | HD=0.5g/0.5g every 12hc | 29−15=200/200/100 every 6hd | 29−15=1g every 8he | |
DH=200/200/100 post-HDd | HD=0.75g every 12he | ||||
Adjustment for liver disease | No | No | No | No | No |
CAZ/AVI: ceftazidime/avibactam; CFD: cefiderocol; CTZ/TAZ: ceftolozane/tazobactam; IMI/CLI/REL: imipenem/cilastatin/relebactam; MERO/VABO: meropenem/vaborbactam.
CTZ is another broad-spectrum cephalosporin already commercially available and administered in combination with a β-lactamase inhibitor (TAZ). CTZ differs from other cephalosporins by its 3- and 7-position side chains with a bicyclic β-lactam/dihydrothiazine ring. The 7-position ring provides activity against GNB, while the 3-position ring increases stability against AmpC and prevents hydrolysis by microorganisms such as P. aeruginosa.16 The combination with TAZ decreases the MIC50-90 of microorganisms producing class A and C β-lactamases such as Escherichia coli, Klebsiella pneumoniae, Enterobacter spp., and Serratia spp. and achieves its activity by irreversibly binding to β-lactamases and undergoing slow hydrolysis. Narrow-spectrum β-lactamases such as TEM-1, TEM-2, SHC-1, and OXA-1 have little effect on CTZ/TAZ activity (Fig. 1). Conversely, BLEE, TEM3-9, SHV-2-4, OXA-2, and CTX-M-3-18 reduce the drug’s activity; however, it may still remain effective. In a review of phase III studies of CTZ/TAZ in complicated urinary tract infection and intra-abdominal infection (IIA) caused by BLEE-producing E. coli and K. pneumoniae, 82% of the 159 pathogens were found to be susceptible and 97.4% were clinically cured.17
CTZ/TAZ maintains its activity against efflux pump-producing bacteria (MexXY, MexAB), in addition to having been found to maintain its activity against several strains of P. aeruginosa with PDR, XDR, and MDR resistance profiles that modify their PBP (penicillin-binding proteins).16–18 However, CTZ/TAZ has no activity against carbapenemases or metallo-β-lactamases, nor does it show any activity against Stenotrophomonas spp. and Acinetobacter spp. (Fig. 1). The pivotal study on the use of CTZ/TAZ in nosocomial pneumonia including VAP19 concluded that it was non-inferior to the comparator (MERO) in a subgroup of patients who could have a better outcome, particularly in the presence of MDR and XDR P. aeruginosa, which is why high-dose CTZ/TAZ is currently a treatment option for VAP caused by resistant micro-organisms, especially P. aeruginosa, which is why in a recent review on the treatment of nosocomial pneumonia20 in a recent expert consensus,20 CTZ/TAZ was included as the first treatment option for VAP with high risk of P. aeruginosa having any kind of resistance profile in the «PANNUCI» algorithm. The differential characteristics between CTZ/TAZ and other modern combinations can be found in Table 1.
It is important to emphasise that, despite the fact that unlike other cephalosporins, CTZ/TAZ has demonstrated great stability against AmpC, the clinical use of this agent has revealed the emergence of resistance during treatment related to AmpC mutations.21–23 The rate of resistance to CTZ/TAZ during administration varies from 3% to 14% and development of resistance during treatment is expected to occur most likely in MDR and XDR isolates, many of which already have one of the required mutations (leading to overexpression of AmpC). When this CTZ/TAZ resistance emerges, susceptibility is generally restored to meropenem and PTZ, which can then be used in treatment.21
Carbapenem+β-lactamase inhibitorMeropenem/vaborbactam (MERO/VABO)The development of new combinations based on «old» carbapenemics, together with new β-lactamase inhibitors that are active on carbapenemases, is one of the most promising strategies to treat carbapenem-resistant Enterobacteriaceae (CRE).
MERO/VABO is the combination of a Group 2 carbapenem (MERO) with a new β-lactamase inhibitor based on cyclic boronic acid (VABO) approved and marketed to treat complicated UTI, intra-abdominal infection, and nosocomial pneumonia, including VAP caused by GNB with limited treatment options. VABO, thanks to its β-lactamase inhibition profile, increases the spectrum of MERO activity to strains of enterobacteria that produce KPC-type carbapenemases and class A carbapenemases.24,25 These enterobacterial strains are typically resistant to other carbapenems, other β-lactams and, in addition, may be resistant to non-β-lactam ATBs due to chromosomal mutations and plasmids.26 Several publications have reported MERO/VABO activity against KPC-producing Enterobacteriaceae to exceed 99%.24,26,27 MERO/VABO is also active against KPC mutant strains, which are resistant to CAZ/AVI (KPC-8, KPC-31) and is capable of decreasing the MIC of strains with diminished susceptibility to MERO due to BLEE or AmpC production (Fig. 1). On the other hand, MERO/VABO is not effective against class B and D carbapenemases. Unfortunately, MERO/VABO activity against Pseudomonas aeruginosa and Acinetobacter spp. is similar to MERO alone; this is because resistance to MERO is mediated by mechanisms that are not affected by VABO, such as membrane permeability, efflux pump dysregulation, and class B and D β-lactamase production.
The second randomised phase III study (TANGO II),28 which evaluated the effectiveness of MERO/VABO relative to the best available therapy (BAT) in patients with various serious infections (including VAP) with suspected carbapenem-resistant Enterobacteriaceae, was terminated prematurely for ethical reasons of efficacy after enrolling only 77 patients. Despite this significantly decreasing the power of the study, an improvement in clinical (59% vs. 26%) and microbiological (65% vs. 40%) cure was observed for patients treated with MERO/VABO over MTD. Specifically, in the VAP subgroup, there was a significant reduction in 28-day mortality (22% vs. 44%) compared to BAT. Even with its limitations regarding the power of the study, TANGO II suggests that MERO/VABO is a suitable treatment option for carbapenemase-producing Enterobacteriaceae. Table 1 illustrates the differential characteristics of MERO/VABO compared to other new combinations.
Imipenem/relebactam/cilastatin (IMI/REL/CIL)IMI/REL/CIL is a combination of a classical carbapenem (IMI), together with a renal dehydropeptidase-I inhibitor (CIL) and a modern β-lactamase inhibitor (REL), which has recently been approved in the European Union for the treatment of nosocomial pneumonia, VAP, complicated UTIs, and ARI in adults with limited treatment alternatives.29,30
REL inhibits class A and C β-lactamases, as well as cephaloporinases produced by Pseudomonas spp. However, REL is not active on class B MBL or class D β-lactamases (Fig. 1).
The addition of REL leads to a marked decrease in the MIC50-90 of IMI on BLEE- and KPC-producing enterobacteria, as well as on IMI-resistant strains of P. aeruginosa.29,31,32 In addition, neither IMI nor REL is subject to bacterial efflux mechanisms; this feature affords it a comparative advantage over other ATBs that are affected by strains expressing efflux pumps.29,32
Although the in vitro activity profile of IMI/CIL/REL on carbapenem-resistant P. aeruginosa strains is similar to CAZ/AVI, IMI/CIL/REL maintains high activity against P. aeruginosa that develop resistance to CAZ/AVI and CTZ/TAZ,33 making it a suitable choice for rescue therapy in severe infections.
IMI/CIL/REL was non-inferior to the comparator PTZ for the primary endpoint of 28-day, all-cause mortality in the randomised RESTORE-IMI 234 study. However, in the pre-defined subgroup analysis of patients with MV or APACHE II of more than 15 points, IMI/CIL/REL presented lower mortality than the comparator.
Although not yet commercialised in our country, the positioning of this combination has yet to be determined with respect to other available therapeutic options with similar characteristics (Table 1); the available evidence suggests that IMI/CIL/REL is an appropriate and well-tolerated treatment option for severe patients (APACHE>15) or in MV affected by severe infections caused by carbapenem-resistant GNB.
Meropenem/nacubactam (MERO/NACU)NACU is a non-β-lactam β-lactamase inhibitor that is administered together with MERO for the treatment of GNB infections. NACU has proven to be active against class A and C β-lactamases and, to a lesser extent, against class D β-lactamases.35,36
On its own, NACU possesses antibacterial activity by binding to PBP2 of several enterobacteria; therefore, when administered in combination with MERO, its effectiveness is increased by binding to other PBPs. This efficacy is observed on BLEE-, AmpC-, KPC-, MBL-, and OXA-48-producing enterobacteria, as well as on AmpC-producing P. aeruginosa strains (derepressed) or BLEE class A type PER or VEB37,38 (Fig. 1). The PK profile of NACU is similar to other inhibitors and the plasma concentration and cumulative urinary excretion is similar to MERO; consequently, MERO/NACU dosing in renal dysfunction is consistent with that established for administration of MERO on its own. These properties make MERO/NACU an outstanding candidate for future clinical research with intractable microorganisms.
Monobactam+β-lactamase inhibitorAztreonem/avibactam (AZT/AVI)AZT is a classic single-ring (monobactam) β-lactam ATB, active against aerobic GNBs, but inactive against gram-positive microorganisms. Its use has been extremely limited in the past due to the high prevalence of BLEE- and AmpC-producing microorganisms. In contrast, MBLs that hydrolyse all β-lactams do not affect monobactams, such as ATZ and this feature has allowed them to be used in certain MBL-producing strains.39,40 However, given that other factors of resistance, such as the class A β-lactamase or AmpC are often present, ATZ can only be used in one out of three subjects with MBL. The addition of AVI, a previously described non-β-lactam β-lactamase inhibitor, restores AZT’s ability to work with MBL-expressing strains, such as VIM (Verona-integron-encoded BML), imipenemases (IMPase), and NDM (New Delhi MBL), along with KPC or other OXA-type carbapenemases.41 ATZ/AVI was more active than MERO, amikacin, and CAZ/AVI in almost completely inhibiting enterobacterial strains with dual carbapenemase production (Fig. 1). However, another study revealed that AZT/AVI was active for all carbapenemase-producing GNB strains, with the exception of NDM-1 and NDM-7 produced by E. coli strains from Singapore.41–44 Its spectrum over all classes of β-lactamases establishes AZT/AVI as a priority in ICUs with high MBL. The appropriate dose in renal dysfunction has yet to be elucidated. Several phase I studies (https://www.withpower.com/trial/phase-1-Renal-Insufficiency-7-2020-bffb2/https://www.clincosm.com/trial/renal-insufficiency-saint-paul-aztreonam-avibactam) may be capable of yielding this information in the near future.
Cephalosporins/β-lactamase inhibitorCefepime/zidebactam (CFP/ZDB)CFP is a well-known fourth-generation cephalosporin that acts by binding to PBP3 and also to PBP1 of gram-positive and gram-negative microorganisms, including P. aeruginosa. AmpC has low affinity for CFP; thus, CFP remains active against enterobacteria with derepressed AmpC. However, CFP can be hydrolysed by some class A β-lactamases, including BLEE, KPC, as well as MBL (class B), in addition to some class D β-lactamases (OXA).45
ZDB is a non-β-lactam ATB with a dual mode of action, involving high and selective affinity for PBP 2 of GNB and inhibition of β-lactamases.46,47 ZDB lends CFP great potential for action against carbapenemase-producing Enterobacteriaceae, P. aeruginosa, and MDR Acinetobacter baumannii, and for those strains of P. aeruginosa that exhibit multiple resistance mechanisms, including the combination of efflux pump stimulation with porin depletion or overproduction of AmpC45,48,49 (Fig. 1). Of particular importance is the marked effect on class D carbapenemase-producing strains and on MBL-producing strains, including VIM, IMP, and NDM, as well as on P. aeruginosa overexpressing AmpC and MBL (VIM and IMI).50
The positioning of CFP/ZDB has yet to be determined with data on clinical use; it will therefore depend on the assigned cut-off point for comparison with other modern ATBs. Until then, and based on in vitro results, it could play a key role in the treatment of patients with MDR enterobacteria and BLEE- and MBL-producing P. aeruginosa.51
Cefepime/taniborbactam (CFP/TBB)TBB is a new β-lactamase inhibitor based on cyclic boronate that lacks antibacterial activity, but is a potent in vitro inhibitor of BLEE and MBL activity.48 TBB imparts CFP with activity against intractable microorganisms including Enterobacteriaceae and MERO-resistant P. aeruginosa, BLEE-producers, AmpC, OXA, KPCs, and MBLs including VIM and NDM52–54 (Fig. 1). Although its in vitro effect is very compelling, clinical studies (CERTAIN-1 in UTI and CERTAIN-2 in VAP) have yet to be undertaken, after which TBB should be positioned with regard to modern MBL inhibitors.
Cefepime/enmetazobactam (CFP/ETZ)ETZ is a penicillinic acid sulphone and a modern β-lactamase inhibitor, especially against BLEE (TEM, SHV, CTX). Combination with CFP provides in vitro activity comparable to MERO, but superior to PTZ against BLEE-producing enterobacteria.55,56 In vitro studies also demonstrate that CFP/ETZ is effective against strains of enterobacteria producing class A, C, and D β-lactamases57,58 (Fig. 1). Clinical data on its effectiveness in VAP compared to other new combinations are still needed to position it clinically.
New cephalosporinsCefiderocol (CFD)CFD is a new siderophore cephalosporin that has been commercialised since 2020 with a dual mechanism of action; in addition to the passive diffusion of CFD through outer membrane porin channels, CFD can bind extracellular free iron through its siderophore side chain. Bacteria naturally secrete siderophores to allow ferric iron to be absorbed from the host environment; as a result, CFD binds to free ferric iron and, therefore, uses the bacterial uptake of siderophores to penetrate the bacterium, a mechanism known as the «Trojan horse».59,60 On the other hand, CFD binds to PFP, especially PFP3, inhibiting the synthesis of the bacterial peptidoglycan cell wall, resulting in lysis and cell death.
CFD is active against all clinically relevant GNBs, such as Enterobacteriaceae, P. aeruginosa, Acinetobacter spp,. as well as Stenotrophomonas maltophilia, yet ineffective against gram-positive cocci (GPCs) or anaerobes61–63 (Fig. 1). The recommended dosages and comparative characteristics are outlined in Table 1. After a single dose of CFD, the half-life of the drug is similar to other β-lactams (2−2.7h), with a volume of distribution of 16–18l. However, protein binding is substantially higher (58%) than CTZ (11%–21%) and CAZ (21%),64 and it is excreted essentially unchanged in urine (60%–90%). Its effect is time-dependent (%T>MIC) and elimination of more than 60% has been observed following a standard session of haemodialysis. Penetration into the lung is good, and in mechanically ventilated patients with VAP, the estimated alveolar fluid (ELF)/free plasma ratio was 0.21 by the end of the 2-g perfusion over 60min and 0.55 two hours after perfusion was complete. ELF penetration was similar in both healthy subjects and ventilated patients, albeit with a decrease below the MIC for the most common microorganisms after 6h; hence, dosing should take place between 4–6h to maintain a%T>CIM greater than 50% between the dosing interval.
The APEKS-NP65 study evaluated the non-inferiority of CFD versus MERO administered as a 3-h extended infusion in 300 patients with nosocomial pneumonia, including VAP, and despite the fact that this form of administration is not contemplated in the guidelines, it is the usual method of administration of MERO. No differences were observed in 14-day mortality (12.4% vs. 11.6%), nor in the frequency of clinical cure (65% vs. 67%) or microbiological eradication (41% vs. 42%) for CFD and MERO, respectively. Finally, the CREDIBLE-CR study was complementary to the previous studies, inasmuch as it examined CFD vs. MTD for the treatment of carbapenem-resistant GNB infections. The study included 150 patients (67 with NP) and no differences in clinical cure (43% vs. 43%) or microbiological eradication (30% vs. 29%) were recorded overall. Interestingly, mortality tended to be higher in CFD (34%) compared to MTD (18%, P=.057), although none of the deaths were attributed to CFD. Given the broad spectrum of action on all BLEE, including MBL, CFD is an ATB that should be available in ICUs with a high frequency of intractable GNB.
Clinical considerations of the use of new antimicrobials in multi-resistance environmentsAppropriate use of the new antimicrobialsThe decision to use or reserve new ATBs is a challenging issue to face. It cannot be properly interpreted if it is not placed in a suitable global context that includes local epidemiology, the characteristics of the patient in particular, the focus of infection to be treated, and, of course, the implementation of antimicrobial optimisation programmes and treatment policies established by protocols agreed by consensus.66 Furthermore, to facilitate the proper use of new antimicrobials, we believe that accurate and early microbiological information is essential. In this respect, modern techniques of early microbiological diagnosis by multiplex PCR67 allow for early and targeted treatment, avoiding overuse of antimicrobials and reducing the selection pressure on the unit. Reserving new antimicrobials and administering alternative combinations of old antibiotics, which may have less of an effect or a higher incidence of side effects, does not appear to be the most appropriate option if rapid techniques are available to rule out the presence of multidrug-resistant microorganisms when choosing empirical or early targeted treatment. In Fig. 2, we suggest a diagnostic and treatment algorithm for ventilator-associated pneumonia that may be helpful to adapt in each ICU, depending on the local epidemiology.
Diagnostic algorithm and positioning of new antimicrobials for the treatment of ventilator-associated pneumonia (VAP).
ATB: antibiotic; BAL: bronchoalveolar lavage; BAS: bronchial aspirate; CPIS: clinical pulmonary infection rating scale; MDR: multidrug-resistant microorganisms; PCT: procalcitonin; CRP: C-reactive protein; PSB: protected brush; rt-PCR: polymerase chain reaction; CXR: chest X-ray; SIRS: systemic inflammatory response syndrome; CT: computed axial tomography.
Continuous or extended perfusion of β-lactam antibiotics has demonstrated to improve the PK/PD conditions of β-lactams by maximising the time during which the plasma concentration remains above the MIC and increasing efficacy. Based on these considerations, modern combinations of β-lactams with an inhibitor should behave in a similar way. Little experience has been published concerning the use of new antimicrobials in extended perfusion. A recent study68 illustrated that the standard dosing of 2g every 8h of CTZ/TAZ was sufficient to obtain plasma levels exceeding MIC (ƒT>MIC) more than 90% of the time, when microorganisms with a MIC<6mg/l were considered. However, only prolonged administration (4h) or continuous infusion of CTZ/TAZ achieved target plasma levels in more than 90% of cases if the MIC of the microorganisms was ≥6mg/l.68 Treatment with a 4-h extended infusion of CAZ/AVI achieved a clinical cure rate of 80% and a microbiological cure rate of 90%.69 In the same study, the authors used a daily dose of 10g CAZ/AVI in 10 patients with severe Pseudomonas aeruginosa and Klebsiella pneumoniae infections, achieving plasma levels of 63.6mg/l, which correlated with high plasma levels/MIC ratio (median 13mg/l) without evidence of neurological involvement or haematological toxicity.
The need to maintain consistently high plasma antimicrobial levels is a particularly relevant issue in critically ill patients, which is why, just as we are doing with other β-lactams, we recommend extended administration of CTZ/TAZ, especially in patients on mechanical ventilation or with increased creatinine clearance.
ConclusionsThe rapid spread of extended-spectrum β-lactamase-producing strains, especially MBL, generally associated with the presence of Enterobacteriaceae or P. aeruginosa, has caused a very serious crisis in healthcare systems.
Increased population mobility, as well as the elevated prevalence of plasmid-mediated resistance emergence, or the high intestinal colonisation by MDR-GNB, contribute to this widespread and uncontrolled spread of resistance around the world.
The clinical importance of these infections is linked to their high mortality, especially since MBL-producing strains are usually resistant to all β-lactams, including new combinations of β-lactams or carbapenemics with β-lactamase inhibitors. In this dire context, the development of new combinations with modern and more potent β-lactamase inhibitors may restore sensitivity to classical ATBs to these treatment-resistant microorganisms. The clinical positioning of each is pending the results of their application in clinical trials in critically ill patients, or compassionate use if the best available treatments fail.
FundingNo funding has been received for this manuscript.
Conflict of interestsAlejandro Rodríguez has received has received fees from PFIZER, MSD, GILEAD, THERMOFISHER, ROCHE, and BIOMEREUX for his participation in educational activities.
The other remaining authors have no conflict of interests to declare.