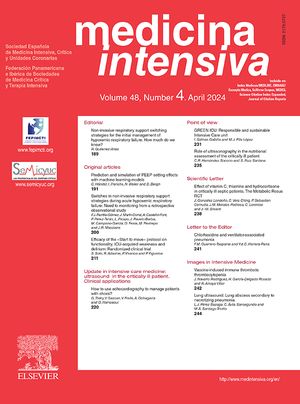
Edited by: Federico Gordo - Medicina Intensiva del Hospital Universitario del Henares (Coslada-Madrid)
More infoAntimicrobial stewardship programs (ASPs) have been shown to be effective and safe, contributing to reducing and adjusting antimicrobial use in clinical practice. Such programs not only reduce antibiotic selection pressure and therefore the selection of multidrug-resistant strains, but also reduce the potential deleterious effects for individual patients and even improve the prognosis by adjusting the choice of drug and dosage, and lessening the risk of adverse effects and interactions. Gram-negative bacilli (GNB), particularly multidrug-resistant strains (MDR-GNB), represent the main infectious problem in the Intensive Care Unit (ICU), and are therefore a target for ASPs. The present review provides an update on the relationship between ASPs and MDR-GNB.
Los programas de optimización del uso de antimicrobianos (PROAs) han demostrado ser una herramienta eficaz y segura que disminuye y ajusta el uso de antimicrobianos en la práctica clínica. El beneficio mostrado es doble, por un parte reduce la presión antibiótica y por tanto la selección de cepas multi-resistentes, y por otro disminuye los potenciales efectos deletéreos en el paciente concreto e incluso mejora el pronóstico al ajustar la elección del fármaco, la posología y los posible efectos adversos e interacciones. Los bacilos Gram-negativos (BGN), especialmente aquellas cepas con mecanismos de resistencia (BGN-MDR), representan el principal problema infeccioso en las unidades de cuidados intensivos y por tanto son el objetivo de los PROAs. En la siguiente revisión actualizaremos aquellos aspectos que combinan y justifican la relación entre los PROAs y los BGN-MDR.
Infection is one of the main causes of admission to the Intensive Care Unit (ICU), and one of the leading factors contributing to complications during admission. Consequently, antibiotic use is essential for the management of critical patients. However, this situation often leads to antimicrobial overuse, with the selection of multidrug-resistant bacterial strains that produces well-known deleterious effects in terms of patient morbidity and mortality, and ICU costs.1,2 Gram-negative bacilli (GNB) are the main cause of infections in the critically ill, and are currently also a leading concern in relation to multidrug resistance problems. The study published by Teshome et al. clearly illustrates the delicate balance among infection, antibiotics and multidrug resistance.3 In 7118 patients with severe sepsis or septic shock, these authors found that each additional day of treatment with an anti-Pseudomonas drug resulted in a 4% increase in the risk of developing de novo resistance. Antimicrobial stewardship programs (ASPs), through a multidisciplinary approach based the experience and knowledge, have been shown to represent a safe and effective tool. The maintenance of these programs is crucial for success, since their suspension leads to a rapid increase in antimicrobial use.4 The present study reviews the real and potential impact of ASPs upon infections caused by GNB - particularly those strains with a multidrug resistance profile.
Effectiveness of ASPsReduction of antimicrobial use and resistancesThe reduction of needless and inappropriate antimicrobial use is the cornerstone of ASPs. Different studies have aimed to correlate the decrease in antibiotic use with the prevalence of colonization and infection caused by multidrug-resistant GNB, with heterogeneous findings among different centers.5–8 It should be noted that the expansion of multidrug-resistant strains is attributable both to the use of antimicrobials and to nosocomial infection control policies. In this regard, it is essential to focus efforts on both aspects in order to avoid both the generation and selection of these strains and their expansion. Nevertheless, despite multiple confounding factors, a systematic review found that after 6 months, most studies on policies for rational antimicrobial use in the critically ill documented a decrease in multidrug-resistant strains in the ICU.9 Several subsequent studies recorded satisfactory outcomes in terms of the reduction of colonization and infection due to multidrug-resistant strains of K. pneumoniae, A. baumannii or P. aeruginosa following the adoption of such programs.10–13 Most of these experiences describe a decrease in resistances secondary to a global reduction in antibiotic use, and although it is difficult to attribute this decrease to a concrete therapeutic drug group, a reduction in the use of carbapenems and quinolones appears to be largely implicated in these findings. It should be mentioned that a reduction in the use of carbapenems appears to have a greater ecological impact on certain species such as Acinetobacter spp. or P. aeruginosa, with a limited effect on carbapenemase-producing Enterobacteriaceae14,15 — though the limited duration of the published studies and their heterogeneity do not allow the drawing of clear conclusions over the long term.
The strategies proposed for optimizing the prescription of antibiotics in the ICU involve successfully implemented restrictive policies, including the postponement of the start of antibiotic treatment in selected patients or restrictions on the use of broad-spectrum antimicrobials.16,17 Such strategies may play a role in the management of non-severe infections, with a relevant impact in terms of decreased global antibiotic use and the selection of resistances to them.
Infection control measures should be integrated with ASPs in order to address the multifactorial problem of multidrug resistance in GNB. In this sense, several studies have described the joint application of antibiotic rationalization and contact isolation measures, environmental decontamination and rectal surveillance cultures, which have been seen to reduce the number of colonizations due to carbapenem-resistant strains.18
Among the ASPs, we consider that a number of aspects are of particular importance in dealing with the problem posed by multidrug-resistant strains (MDR-GNB): dosing of the antibiotic, combination antibiotic treatment, de-escalation, the positioning of new antibiotics, the duration of treatment, and the microbiological diagnosis.
Antibiotic dosing, PK/PD optimizationIt is known that antibiotic dosing in many patients admitted to the ICU is suboptimal due to significant changes in the pharmacokinetics of the drug – particularly the volume of distribution and renal clearance. The important inflammatory processes, high fluid administration and use of organ-support measures – including renal replacement therapy and extracorporeal membrane oxygenation (ECMO) – in the context of critical care complicate adequate dosing because of the increased pharmacokinetic variability observed in such patients.19
Many studies have evidenced the importance of the individualized adjustment of antibiotic dosing to improve the clinical outcomes in the critically ill, based on pharmacokinetic variability and the susceptibility of the pathogens causing infection, as determined from their minimum inhibitory concentrations (MICs).20–23 With regard to time-dependent antimicrobials, many publications warrant the use of betalactams in extended or continuous infusion versus intermittent infusion in seriously ill patients, individuals with high glomerular filtration rates, or patients infected with bacteria highly resistant to antimicrobials.24,25 In relation to concentration-dependent antibiotics, the increase in the volume of distribution and the increase in MIC of the bacteria are key factors in determining the dosage of such drugs. The identification of patients at risk of underdosing should be a priority for ASPs. A recent therapeutic position statement on antimicrobial monitoring in critical patients published by a number of scientific societies reflects the main pharmacokinetic/pharmacodynamic (PK/PD) parameters to be established in this group of patients,26 and constitutes a reference document for the work of ASP teams in optimizing dosing in the ICU. It should be mentioned that the PK/PD ratio may vary not only between different antibiotics of the same drug family but also depending on the bacterium causing the infection. Table 1 summarizes the PK/PD cut-off points to be reached for the main antimicrobials used in the critically ill.
PK/PD targets in antimicrobial dosing in critical patients.
Antibiotic | PK/PD target |
---|---|
Aminoglycosides | Cmax/MIC≥8–10; AUC 0–24/MIC ≥ 110 |
Carbapenems | 50–100% fT>MIC |
Cephalosporins | 45–100% fT>MIC |
Penicillins | 50–100% fT>MIC |
Fluoroquinolones | AUC 0–24/MIC≥125–250; Cmax/MIC ≥ 12 |
Colistin | AUC 0–24/MIC: 6.6−13.7 |
Tigecycline | AUC 0–24/MIC>6.96a; AUC(0–24)/MIC>17.9b |
Fosfomycin | 70% fT>MIC; AUC 0–24/MIC > 24 |
The need to guarantee early and adequate treatment for critical patients with infections in a multidrug-resistant environment implies that many patients receive broad-spectrum antibiotic therapy. However, in many cases the antibiotic treatment can be reduced once the results of the microbiological tests become known, and in the absence of multidrug-resistant pathogens. Due to the increase in resistances to broad-spectrum antimicrobials (carbapenems, aminoglycosides, colistin, ceftolozane/tazobactam or ceftazidime/avibactam), the use of such drugs should be limited to patients with a strong suspicion of infection caused by multidrug-resistant strains sensitive to these antimicrobials - with minimization of the duration of treatment. On the other hand, since fluoroquinolones have been widely associated with the appearance of resistant strains, ASP teams should seek to limit their use in critical patients to concrete situations only.27
Despite the abundant evidence that antibiotic de-escalation is not associated with poorer clinical outcomes28,29 and the fact that the clinical guides recommend its use,30 de-escalation is not a standard practice in our ICUs. De-escalation is not without difficulties and aspects that need to be taken into account. For example, the ASP teams must inform intensivists that the use of third-generation cephalosporins for the treatment of infections due to Enterobacteriaceae with inducible betalactamases (Enterobacter, Proteus, Morganella, or Serratia spp.) may give rise to resistant strains and thus to treatment failure. On the other hand, the impact of antibiotic de-escalation on the selection of multidrug-resistant GNB remains subject to controversy. In this regard, recent studies have found that cephalosporins may have a greater impact on the microbiota than drugs such as piperacillin/tazobactam.31,32 Nevertheless, de-escalation is considered to be largely beneficial for drugs such as carbapenems, aminoglycosides or quinolones, since a decrease in their use has been associated with lesser infection/colonization by multidrug-resistant strains.
Combination antibiotic treatmentIn recent decades, combination therapy has been advocated as a tool for improving clinical outcomes, in view of data indicating its greater bactericidal activity in vitro. The use of combination antibiotic treatment in infections caused by multidrug-resistant GNB in critical patients has become common practice in many centers. Nevertheless, such use remains controversial and debatable, with no clearly favorable results to be found in the literature even in neutropenic patients.33–37 The advantages of combining antibiotics with different mechanisms of action include a broadening of the antibacterial spectrum in empirical treatments, theoretical synergic effects, and a decrease in the risk of resistances developing during treatment. However, it is important to note that combination therapy likewise has not been correlated to date to any lesser generation of resistances.36 In addition, its use requires several precautions related to a greater toxicity risk, the selection of resistant strains, and cost considerations. Combination therapy as guided management therefore should be reserved for concrete situations in which the new betalactams administered as monotherapy do not suffice to resolve the infection.
New antibioticsOver the last decade there has been an increase in the number of antibiotics that are effective in treating infections due to multidrug-resistant GNB strains, and which involve different mechanisms of action – including new betalactamase inhibitors and cephalosporins with potent activity against these bacteria.38 However, we have also witnessed the rapid appearance and publication of strains resistant to these new antibiotics.39,40 In this respect, ASP teams must monitor emergent resistances to these new drugs and include them among the local susceptibility data, in order to continuously assess which drug maintains the best activity with the absence of cross-resistances. The local epidemiology must condition the decision as to which antimicrobial should be used at each center, in accordance with the most frequent mechanism of resistance to carbapenems (KPC, OXA-48, MBL) in each Unit. In addition, since the resistance mechanisms are complex and are often of a combined nature, Microbiology Departments should provide guidance as to which strains should be tested for sensitivity to these new drugs. On the other hand, although a given agent may be preferred for empirical use based on the local epidemiology, the treatment decisions should be analyzed by the team based on the characteristics of the patient and the pathogen involved. Avoiding prescription errors with these drugs (indication, dosage or duration of treatment) is crucial in order to minimize the development of resistant strains, and constitutes one of the principal responsibilities of the ASP teams.
Duration of treatmentThe Surviving Sepsis Campaign (SSC) guides establish the general recommendation of 7–10 days of antibiotic treatment in patients with sepsis or septic shock.41 Special consideration applies to cases in which the infection has not been resolved, in the case of concrete etiologies, or in situations of neutropenia. On the other hand, infections such as pyelonephritis or spontaneous bacterial peritonitis can be managed with fewer days of antibiotic treatment.42 In the specific case of infections due to GNB, the conditions are not very different. In ventilator-associated pneumonia, 8 days of treatment has been found to be as effective and safe as 15 days of treatment. However, infections due to non-fermenting GNB such as Pseudomonas spp., Acinetobacter spp. or Stenotrophomonas spp. could be associated to a greater number of recurrences with the short treatment regimen.43,44 In bacteremia due to GNB with clinical stability by day 7 of treatment, no added benefit in terms of mortality, clinical response or hospital stay is to be expected by extending therapy to 14 days.45,46 Likewise, intraabdominal infection with a surgically controlled focus can be safely managed with 8 days of treatment versus the usual duration of 15 days.47
Information on the duration of treatment, and referred to extrapolation of the aforementioned results, in infections produced by MDR-GNB, is limited. However, resistance to antibiotics is not necessarily accompanied by more virulence mechanisms (toxins, biofilm formation, etc.) – indeed, the opposite often applies – and the difficulties found in treating such infections was due to the few effective antibiotics available, and especially to their characteristics. Drugs such as colistin, fosfomycin or even the aminoglycosides have deficiencies in terms of pharmacokinetic control and safety problems that limit their dosification and indication. Nevertheless, with the introduction of the new molecules, affording efficacy and safety in the treatment of infections produced by MDR-GNB, we can consider using the same treatment duration schemes.48Table 2 shows some of the published recommendations on the duration of antibiotherapy in infections due to GNB, though the individualization of treatment based on the clinical course must be contemplated by the ASP teams.
Recommended duration of antimicrobial therapy in critical patients.
Days of treatment | References | |
---|---|---|
Community-acquired pneumonia | 5−7 days | 60 |
Ventilator-associated pneumonia | 7 days | 43,44 |
Ventilator-associated pneumonia due to non-fermenting GNB (P. aeruginosa, A. baumannii, S. maltophilia) | 14 days | 43,44 |
Catheter-associated bacteremia | 10−14 days | 45,46 |
Urinary infection | 5−7 days | 42 |
Intraabdominal infection | 8 days | 61 |
The development and implementation of rapid identification tests in critical samples (blood cultures, respiratory samples or cerebrospinal fluid) has represented a great step forward in the early management of infections due to multidrug-resistant GNB in the critically ill. These techniques allow the early optimization of antimicrobial treatment, shortening the time to the start of adequate therapy, and having a potential impact upon patient morbidity and mortality.49 These techniques are based on a number of principles: from disc diffusion methods that analyze sensitivity directly from positive blood cultures to more sensitive and faster molecular tests that allow the detection of bacterial growth from clinical samples – providing results within a few hours.50–52 A very important contribution has been the development of molecular techniques for the detection of resistance genes.53 In this regard, the introduction of rapid carbapenemase detection tests has made it possible to successfully implement early treatment optimization programs, with promising results.54 Nevertheless, these tests require expertise on the part of the ASP teams in order to guarantee efficiency in a scenario characterized by resource limitations. Table 3 summarizes the principal characteristics of the main rapid bacteremia diagnostic techniques.
Principal characteristics of different rapid bacteremia diagnosing systems.
FilmArray (Biomerieux) | Verigene System (Luminex Nanosphere) | QuickFISH (AdvanDX) | ePlex BCID (Gen Mark DX) | Sepsis Flow Chip Assay (Master Diagnostica) | Magicplex Sepsis (SeeGene) | |
---|---|---|---|---|---|---|
Type of test | PCR multiplex | PCR multiplex | PCR multiplex | PCR multiplex | PCR multiplex | Endpoint/real-time PCR |
Clinical sample | Blood culture (+) | Blood culture (+) | Blood culture (+) | Blood culture (+) | Blood culture (+) | Blood |
Pathogens detected | 24 pathogens: GN: 11 GP: 8 Candida: 5 Genes: mecA, vanA/B, KPC | 2 panels: 1) GN: 9 + Genes: CTX-M, IMP, KPC, NDM, OXA, VIM 2) GP: 13 + Genes: mecA, van A/B | 10 pathogens: GN: 3 GP: 4 Candida: 3 | 3 panels: 1) GN: 21+ Genes: CTX-M, IMP, KPC, NDM, OXA, VIM 2) GP: 20 + Genes: mecA/C, van A/B 3) 16 Fungi | 18 pathogens: GN: 10 GP: 7 C. albicans + 20 resistance genes | 91 pathogens: GN: 12 GP: 73 + Genes: mecA, vanA/B 6 Fungi |
Time to result | 1h | 2.5 h | 0.5 h | 1.5 h | 3 h | 6 h |
GN: Gram-negative bacteria; GP: Gram-positive bacteria.
In recent years, artificial intelligence (AI) and machine learning techniques have produced new tools for predicting the risk of colonization and infection by multidrug-resistant bacteria, and the development of resistances associated to antibiotic use.55 Such techniques have also been used to develop supporting tools capable of learning classification standards in order to label antibiotic prescriptions as inappropriate and to recommend both dose adjustments and antibiotic suspension and de-escalation.56 The enormous potential of these tools makes it essential to continue exploring their usefulness in routine clinical practice over the coming years.
ASP indicators for Gram-negative bacteriaMonitoring antibiotic use in the ICU is a core element in ASPs. Traditionally, the defined daily dose (DDD), weighted by the number of stays, has been the most widely used parameter. However, the advisability of employing DDD as sole indicator is questionable. In effect, indicators based on DDD may overestimate antibiotic pressure in situations where greater than the defined doses are used – without this implying a greater risk of selection or induction of resistances (indeed, it may even contribute to avoid them). This is of growing importance, since in recent years the information provided by PK/PD studies has evidenced the need to administer higher doses for certain multidrug-resistant microorganisms or in seriously ill patients with increased renal clearance.57 Therefore, epidemiological changes or modifications of the dosing recommendations may have a decisive impact upon the indicators. In recent years, more specific drug use indicators have been proposed to measure the utilization of antimicrobials.58Table 4 describes some of them.
Indicators proposed in ASPs in multidrug-resistant gram-negative infections.
Indicator | Calculation | Justification |
---|---|---|
Consumption indicators | ||
DDD/100 stays | ||
Total | Suma DDD/100 stays | Most widely used indicator in the literature for evaluating impact of ASPs. Justified by the relationship between antibacterial use and the selection and spread of bacterial resistances |
Carbapenems | Sum DDD/100 stays of carbapenems (imipenem, meropenem and ertapenem) | Broad-spectrum agents. Their use can indicate activity spectrum abuse. High ecological impact |
Aminoglycosides | Sum DDD/100 stays of aminoglycosides (amikacin, gentamycin, tobramycin) | |
Quinolones | Sum DDD/100 stays of quinolones (ciprofloxacin, levofloxacin, moxifloxacin) | |
Tigecycline | Sum DDD/100 stays of tigecycline | |
Colistin | Sum DDD/100 stays of colistin | |
New antibiotics | Sum DDD/100 stays of ceftolozane-tazobactam and ceftazidime-avibactam | Reserve agents for documented infections due to microorganisms resistant to the rest of betalactams. Economic impact |
Ratio Amoxicillin/clavulanate/piperacillin/tazobactam | Numerator: DDD/100 stays of amoxicillin-clavulanate Denominator: DDD/100 stays of piperacillin-tazobactam | Promote the use of amoxicillin-clavulanate in infections where it is not necessary to resort to drugs with activity against Pseudomonas |
Ratio Ertapenem/Meropenem+Imipenem | Numerator: DDD/100 stays of ertapenem Denominator: DDD/100 stays of meropenem and imipenem | Promote the use of ertapenem in infections where it is not necessary to resort to drugs with activity against Pseudomonas |
Diversification of anti-Pseudomona betalactams | Heterogeneity index of DDD/100 stays of anti-Pseudomonas carbapenems, piperacillin-tazobactam and anti-Pseudomonas cephalosporins and aztreonam | The more diverse the use of these betalactams, the less the antibiotic pressure upon each group of them |
Treatment optimization indicators | ||
% Appropriate empirical treatment | Number of patients with treatment suited to result of culture/total number of patients with infection | Evaluates the suitability of empirical treatment in nosocomial infection, allowing its adjustment in the event of negative results secondary to changes in local epidemiology |
Sepsis | ||
VAP | ||
UTI | ||
CAB | ||
Empirical antibiotic treatment according to local guides | Number of patients with empirical treatment according to local guides/number of patients with infection | Evaluates the suitability of empirical treatment to the local treatment guides |
Microbiological indicators | ||
% Nosocomial infections caused by multidrug-resistant strains | Number of strains causing multidrug-resistant nosocomial infections/total number of nosocomial infections | Evaluates the resistance profile (total and to key antibiotics), to determine the local epidemiology and optimize the empirical treatment guides |
% Resistance to target antibiotics | Number of strains causing nosocomial infections resistant to target antibiotics/total number of nosocomial infections |
DDD: Defined daily dose; VAP: Ventilator-associated pneumonia; UTI: Urinary tract infection; CAB: Catheter-associated bacteremia.
It is essential to complement drug use indicators with other parameters for assessing prescription quality, despite the known difficulties involved in evaluating their suitability.59–61 Quality assessment must be made based on individualized analyses among different professionals, since they may help in identifying targets for intervention and improvement. The most commonly used indicators have been the suitability of empirical treatment according to the guides, and the time to start of adequate antimicrobial treatment or the switch from intravenous to oral therapy.
With regard to the microbiological indicators, it is necessary for the Microbiology Department to participate in periodic sessions on drug resistances in the ICU, in order to allow the magnitude of the problem to be known, underscore the importance of ASPs, and address the results of the infection prevention programs, the state of possible epidemic outbreaks, and points for improvement.
Lastly, in a context of limited human resources and time, it is necessary to equip hospitals with electronic systems allowing better and faster measurement of indicators, including not only DDD but also the prescribed daily doses, as well as the empirical treatment used in concrete infections - allowing rapid and accessible evaluation of the ASP activities and important time savings in the identification of prescriptions amenable to intervention.
ConclusionsIntensive Care Units face a situation of endemic multidrug-resistant GNB infections. The introduction of new drugs with activity against these pathogens offers hope, though it is crucial to guarantee their appropriate use in order to limit the risk of generating resistances. Despite the introduction of a number of rapid phenotype tests for detecting carbapenemase-producing GNB strains, rapid genotype tests are still needed in order to precisely delimit the resistance mechanisms.
The application of effective interventions on the part of the ASP teams is extremely important, and requires leadership by experts in infectious diseases, the incorporation of training targets, collaboration among the teams that intervene in the administration of antimicrobials, the optimization of doses, the promotion of de-escalation according to the microbiological data and the situation of the patient, and the continuous monitoring of resistances within the Unit.